Abstract
Purpose
The purpose of this paper is to develop a method suitable for the design of reinforced concrete columns subjected to a standard fire.
Design/methodology/approach
The Zone Method – a ’simplified calculation method” included in Eurocode 2 – has been developed by Hertz as a manual calculation scheme for the check of fire resistance of concrete sections. The basic idea is to disregard the thermal strains and to calculate the resistance of a cross-section by reducing the concrete cross-section by a “damaged zone”. It is assumed that all fibers can reach their ultimate, temperature dependent strength. Therefore, it is a plastic concept; the information on the state of strain is lost. The calculation of curvatures and deflections is thus only possible by making further assumptions. Extensions of the zone method toward a general calculation method, suitable for the implementation in commercial design software and using the temperature dependent stress–strain curves of the Advanced Calculation Method, have been developed in Germany. The extension by Cyllok and Achenbach is presented in detail. The necessary assumptions of the Zone Method are reviewed, and an improved proposal for the consideration of the reinforcement in this extended Zone Method is presented.
Findings
The principles and assumptions of the Zone Method proposed by Hertz can be validated.
Originality/value
An extension of the Zone Method suitable for the implementation in design software is proposed.
Keywords
Citation
Achenbach, M. and Morgenthal, G. (2016), "Extension of the Zone Method of Eurocode 2 for reinforced concrete columns subjected to standard fire", Journal of Structural Fire Engineering, Vol. 7 No. 2, pp. 82-96. https://doi.org/10.1108/JSFE-06-2016-007
Publisher
:Emerald Group Publishing Limited
Copyright © 2016, Marcus Achenbach and Guido Morgenthal. Published by Emerald Group Publishing Limited.
License
This article is published under the Creative Commons Attribution (CC BY 3.0) licence. Anyone may reproduce, distribute, translate and create derivative works of this article (for both commercial and non-commercial purposes), subject to full attribution to the original publication and authors. The full terms of this licence may be seen at http://creativecommons.org/licences/by/3.0/legalcode
1. Introduction
The Zone Method for the check of fire resistance of reinforced concrete cross-sections is part of EN 1992-1-2 (2004b). It was developed by Hertz (1985) as a scheme for a manual calculation. The concept is to reduce the effective dimensions of the cross-section to simulate the mean deterioration of the concrete. It is assumed that the remaining cross-section can be modeled with a constant concrete strength. Therefore, simplifications and assumptions are necessary: thermal strains are disregarded; the resistance of the cross-section is calculated with a reduced cross-section and simplified material properties; and the ultimate buckling load of a column is determined with Rankine’s formula.
The description of the Zone Method in EN 1992-1-2 (2004b) is incomplete, especially for concrete columns. It is not possible to calculate the fire resistance without background information, as given by Hertz (1985). Hence, the use of the Zone Method in Germany is only allowed by building authorities, if the comments and interpretations by Zilch et al. (2010) or Cyllok and Achenbach (2009) are considered. Both papers are listed as non-contradictory complementary information (NCI) in the German National Annex (DIN EN 1992-1-2/NA, 2010).
These interpretations develop the Zone Method to a general method similar to EN 1992-1-1, 5.8.6 (2004a) with a reduced cross-section and temperature dependent material properties. Zilch, Müller and Reitmayer propose to consider the effect of thermal strains by shifting the stress–strain curves for steel and concrete. Cyllok and Achenbach suggest to reduce the strength of the compressed reinforcement.
The intention of both advancements is to establish an extended Zone Method, which can be easily implemented in commercial software for the design of concrete columns. In contrast to the Advanced Calculation Method, the Zone Method does not need a detailed temperature distribution of the cross-section for the mechanical analysis, as the concrete zone is modeled with constant temperature and material properties. This makes it easy to adjust commercial software for the design of the fire resistance, a coupling of the thermal and mechanical analysis of the cross-section is not required. For example, the temperatures for the centroid and the reinforcement at a given fire resistance can be taken from figures displayed in EN 1992-1-2 (2004b), Annex A.
The benefit of an extended Zone Method is its suitability for the “hot” design of reinforced concrete columns. This method follows the same principles which are used for the design at room temperature, mainly a concrete cross-section with constant material properties. The purpose of an extended Zone Method is the determination of the required area of reinforcement.
For a calculation with the Advanced Calculation Method the area of reinforcement must be known a priori. The result of the calculation is a fire resistance time for a given heating curve or an ultimate load for a fixed time step of a given heating curve. So it is suitable for the recalculation of laboratory tests or existing structures.
In Section 2, the basic assumptions and principles of the Zone Method are presented. Section 3 shows the extensions by Cyllok and Achenbach and gives information on recalculation of fire tests. In Section 4, the assumptions of Section 2 are reviewed. A proposal for the extension of the Zone Method to a general calculation method is given in Section 5. Finally, one worked example is presented in Section 6.
2. Assumptions of the Zone Method for compression members
Hertz (1985) assumes that the thermal strains of heated concrete cross-sections can be recovered by transient thermal strains. The explicit creep model by Anderberg and Thelandersson (1976) is used by Hertz for proof. The transient thermal strains εtr are described here as follows:
where σ = stress; fck = strength of the concrete at 20°C; and εth= thermal strain.
At a stress level |σ|/fck ≥ 0.43 thermal strains can be fully recovered by transient thermal strains. Therefore, Hertz concludes that the thermal strains can be disregarded.
In Figure 1, a concrete wall, heated on both sides, is displayed. It is assumed that the isotherms are parallel to the surface, and that all fibers achieve their ultimate strength. The resistance of the concrete wall per unit length NR,c,fi is as follows:
where h = height of the wall; kc(θ) = fc,θ/fck; and fc,θ = concrete strength at temperature θ.
The parameter kc,m denotes the mean strength of the concrete:
The reduced mean strength of the concrete is represented by a minimized cross-section with an equivalent resistance. The strength at the center M is used for reference:
where θM = temperature at the center M.
The relative height ηf of the reduced cross-section is calculated by:
The width of the “damaged” zone az,f is given by:
The resistance of the reinforcement NR,s,fi is calculated using the strength at 0.2 per cent proof strain:
where As = total area of reinforcement; ks,0.2% = fs,0.2%,θ/fyk; fs,0.2%; θ = reinforcement strength at temperature θ for 0.2 per cent proof strain; and fyk = reinforcement yield strength at 20°C.
It must be mentioned that Hertz (1985) uses simplified equations for the reduction factors kc(θ) and ks,0.2%(θ). The factors given in EN 1992-1-2 (2004b) are not based on simplifications – they are derived from the stress–strain curves supplied for the Advanced Calculation Method.
Hertz states that the peak strain εc1,θ corresponding to fc,θ can be approximated by |εc1,θ|≈3.5%/kc(θ). Reducing the strength of the concrete by kc(θ) and increasing the peak strain εc1,θ by 1/kc(θ) leads to the following:
where Ec = modulus of elasticity at 20°C.
Under the assumption that all fibers are under constant compression, the mean stiffness of the concrete wall EIc,fi can be calculated by:
The reduced mean stiffness is represented by an equivalent cross-section with the material properties at the center M:
where θM = temperature at the center M.
The relative height of the cross-section with equivalent stiffness ηEI is given by:
The “damaged” zone az,EI for a cross-section of equivalent stiffness is given by:
The calculation of equation (11) can be laborious and is not suitable for a manual calculation scheme. Therefore, Hertz proposes a simplified empiric equation:
Equation (12) can be rewritten using equations (13) and (5) as follows:
According to Hertz, the reduction of the strength of the reinforcement is proportional to the reduction of the modulus of elasticity:
where Es = modulus of elasticity at 20°C.
For a centrically loaded wall with pin ended conditions, the Euler buckling load for concrete Nb,c,fi and steel Nb,s,fi can be calculated as follows:
where lcol = length of the wall; EIc,fi = bending stiffness according to equation (10); zs = lever arm of reinforcement; and As = total area of reinforcement.
The ultimate load Nu,fi of the column according to Hertz is given by Rankine’s formula:
Where NR,c,fi and Nb,c,fi are both calculated using az,EI as “damaged” zone.
3. Extension by Cyllok and Achenbach
Richter and Zehfuß (1999) compared the results of the Zone Method with those of the Advanced Calculation Method for bending members. The calculated moment resistance of the Zone Method has always been lower compared to the Advanced Calculation Method. Therefore, the use of the Zone Method for bending members is allowed according to the German National Annex to EN 1992-1-2 (DIN EN 1992-1-2/NA, 2010).
The use of the Zone Method for concrete compression members has been discussed controversially in Germany. The description of the Zone Method for columns in the Eurocode (EN 1992-1-2,2004b) is incomplete, which led to misunderstandings and misinterpretations. In particular, it has not been considered that Rankine’s formula – as given in equation (18) – is used for the calculation of the ultimate load in the Zone Method.
Cyllok and Achenbach (2009) examined three interpretations of the Zone Method and made a proposal for an extension. The aim and purpose of this extension was the development toward a general method according to EN 1992-1-1 (2004a) with only slight modifications of the Zone Method as described in Section 2. All research done for bending members, e.g. from Richter and Zehfuß (1999), should still be valid for an extended Zone Method.
The proposed calculation scheme for a square concrete column is represented in Figure 2, left hand side. The assumptions are as follows:
Thermal stresses are neglected.
The cross-section is reduced by az,EI.
The concrete is represented with constant material properties from the Advanced Calculation Method at the temperature θM.
The peak strain of the concrete |εc1,θ| is at least 3.5‰.
The reinforcement is considered using the material properties from the Advanced Calculation Method.
The strength of the compressed reinforcement is reduced by Δσ=2‰ · Es(θs).
The Assumptions 1 to 5 are in accordance with Hertz because the material properties for simple calculation methods supplied in the Eurocode (EN 1992-1-2 (2004b)) are derived from the stress–strain curves for the Advanced Calculation Method. The effect of Assumption 6 compared to the Advanced Calculation Method has been examined by Cyllok and Achenbach (2009) for three columns. The shapes of the calculated moment-curvature diagrams are comparable to those of the Advanced Calculation Method. The ultimate load for a given fire resistance deviates +20/−18 per cent from the Advanced Calculation Method.
Laboratory tests carried out at TU Braunschweig and documented in the thesis of Haß (1986) have been recalculated by Cyllok and Achenbach (2011) using this extended Zone Method. The mean ratio of calculated time to failure to experimental time to failure η = tcal /texp is 0.904 with a standard deviation σ = 0.218. Haß (1986) also recalculates these 47 columns with an Advanced Calculation Method and obtains a mean of μ=0.966 and standard deviation of σ = 0.207. Hence, the results from Cyllok and Achenbach (2011) are comparable and the proposed method can be regarded as safe and accurate.
The proposed extension of the Zone Method by Cyllok and Achenbach (2009) is mentioned as NCI in the German National Annex (DIN EN 1992-1-2/NA, 2010) to EN 1992-1-2 (2004b) and can be used for the design of columns subjected to fire. But the reduction of the compression strength is chosen empirically, which has been criticized by Gelien (2011). Also, the exact limits of this approximation compared to the Advanced Calculation Method have not been determined yet. Furthermore, the Zone Method itself makes many assumptions, which have not been proved with the material laws given in EN 1992-1-2 (2004b).
4. Proof of the assumptions
The basic ideas of the Zone Method are that thermal strains can be disregarded and that each fiber of the cross-section can achieve its ultimate strength. This means, it is a plastic concept. It cannot be proven analytically – it can be validated by comparing the results with the Advanced Calculation Method. Verification is only possible by the recalculation of laboratory tests.
Therefore, the stress distribution in a wall, considering different material models, is examined. The material models from EN 1992-1-2 (2004b), Anderberg and Thelandersson (1976) and Gernay and Franssen (2012) are used for the Advanced Calculation Method. In the Eurocode model (EN 1992-1-2 (2004b)), the effects of transient thermal strains are included in the stress–strain relationship for concrete (implicit creep). Anderberg and Thelandersson (1976) use an empirical creep function, which has been developed from their own laboratory tests. Gernay and Franssen (2012) also use an empirical creep function. It is based on the material parameters given in ENV 1992-1-2 (1995). Both latter material models use an explicit creep function, which is able to represent stress and temperature dependent stress redistributions.
The stress distribution is displayed in Figure 3 for an axial force per unit length of −0.35 · NRd and −0.70 · NRd with:
where Ac,net = net concrete cross-section; α = 0.85; fck = concrete strength at 20°C; γc = 1.5; As,tot = total area of reinforcement; fyk = steel yield strength at 20°C; and γs = 1.15.
The effect of the stress redistribution is evident for the explicit creep models. As expected, it is more distinctive for higher compression forces. Hence, the assumption of Hertz seems to be reasonable, though it cannot be proven in general. It must also be mentioned that the reinforcing steel hinders the transient thermal strains of the concrete fibers, which has not been fully considered by Hertz.
Hertz states that the axial resistance of a cross-section can be calculated with a reduced concrete cross-section [equation (4)] and the 0.2 per cent proof strength of the reinforcement [equation (7)]. The axial resistance of walls using the method of Hertz and the Advanced Calculation Method with the material models from Eurocode, Anderberg and Thelandersson, Gernay and Franssen is calculated for the parameters given in Table I. The net concrete cross-section has been considered in all calculations. The height of the “damaged” zone for the Zone Method has been calculated with equation (14) because the reduction of stiffness is decisive for compressed walls.
The results are displayed in Figure 4. For each examined cross-section, the result for the Advanced Calculation Method using material models from Eurocode is plotted on the y-axis. The result of the other models is plotted on the x-axis. All results are above the bisecting line, i.e. the Eurocode model gives the highest axial resistance. The results from the Zone Method are close to the results of the Advanced Calculation Method with the Eurocode material model. Therefore, the calculation of the axial resistance with the Zone Method can be considered as validated for walls.
The peak strains εc1,θ corresponding to fc,θ are plotted in Figure 5 for the considered material models. The approximation |εc1,θ|≈3.5‰/kc(θ) matches the peak strains of the explicit creep models up to a temperature θ≈700°C. It is obvious that the peak strains of the implicit creep model of the Eurocode are much larger.
The proposed simplification given in equation (8) can be validated by comparison with the explicit creep models. The ratio kEc(θ)=Ec,fi(θ)/Ec,fi(20°C) is pictured in Figure 6 for the considered material models. The proposed approach kEc(θ)≈(kc(θ))2 is closer to the results from Gernay and Franssen, where the model from Anderson and Thelandersson gives lower modulus of elasticity. As expected, the implicit material model of the Eurocode gives the lowest results.
The calculation of the “damaged” zone az,EI should also lead to comparable results for each of the considered explicit creep models. The results for az,f [equation (6)], az,EI [equation (12)] and the related simplification given in equation (14) is calculated for walls with a height between 10 and 60 cm. The results are displayed in Figure 7. The evaluation of equations (12) and (14) leads to almost identical results. For h≥30 cm the calculated az,EI is nearly constant. Equations (8)-(12) are also evaluated for the modulus of elasticity for the models from Anderberg and Thelandersson and Gernay and Franssen: the simplification given in equation (8) is replaced by Ec,θ of the corresponding model. Both models lead to larger “damaged” zones, the results seem not to be constant for “thick” cross-sections.
The derivation of the damaged zone az,EI is only valid under the assumptions that the isotherms are parallel to the surface, and all fibers are under constant compression. These assumptions are violated for columns heated on four sides because the isotherms are not parallel. They are even more violated for columns with a large eccentricity because the stress distribution is not constant for the cross-section. For columns, proof can only be given by recalculation of laboratory tests and by the comparison with the Advanced Calculation Method of calculated moment-curvature diagrams. It must be mentioned that the tested columns (Haß, 1986) cover only cross-sections 20 × 20 cm (14 tests), 30 × 30 cm (32 tests) and 30 × 40 cm (1 test). Interpreting Figure 7 within the mainly tested range 20 cm ≤ h ≤ 30 cm confirms that the simplified calculation of az,EI given in equation (14) is of sufficient accuracy for a simplified calculation method.
5. Proposal for an extension
As shown in Section 4, the axial resistance of a wall calculated with the Zone Method is close to results calculated by the Advanced Calculation Method, if the resistance of the reinforcement is calculated using the 0.2 per cent proof strength. If the Zone Method for manual calculation is extended toward a general method, suitable for a calculation by computer, the ultimate strength of the concrete fc,θ and reinforcing steel fs,θ=ks,0.2% ·fyk are replaced by the stress curves given by the Advanced Calculation Method. The new proposal is to reduce the strength of the reinforcement by ηs. The resistance of the reinforcement is calculated by:
where As = area of reinforcement; ηs (θ) = reduction factor for reinforcing steel; and σs(θ,ε) = stress of reinforcing steel.
The parameter ηs can be interpreted as a reduction of the area of reinforcement, analogous to the factors ηf and ηEI for the concrete cross-section. This concept was also proposed by Holmberg and Anderberg (1993) for the 500°C isotherm method. They proposed to reduce the area by 50 per cent, if only four corner bars are present. For more reinforced columns, the corner bars are neglected.
Regarding the results for the axial strength given in Section 4, the limitation to the 0.2 per cent proof strength gives conservative results compared to the Advanced Calculation Method. The results for the ratio fs,0.2%(θ)/fs,y(θ), where fs,y(θ) = yield strength of reinforcement, are displayed in Figure 8 for cold worked steel.
Cyllok and Achenbach proposed to reduce the compression strength of the reinforcing steel by Δσ=2% · Es(θs). This approach has been proofed by the recalculation of laboratory fire test, as explained in Section 3. The reduced compression strength σs,red and the yield strength fsy,red are given by:
where σs(θ,ε) = stress of reinforcing steel; Es(θ) = tangent modulus of elasticity of reinforcing steel; and fsy(θ) = reinforcement yield strength at temperature θ.
The ratio |fsy,red(θ)|/|fsy(θ)| is also plotted in Figure 8. It is obvious that the results are conservative for temperatures colder than 400°C: at room temperature only 20 per cent of the yield strength is considered. For temperatures above 400°C, the relative strength is almost constant with a value of 50 per cent of the yield strength at elevated temperatures.
It is proposed to use the following reduction factor ηs (θ) for the reinforcing steel:
where θ = temperature of the reinforcement (°C).
The reduction factor ηs (θ) is also displayed in Figure 8. The effect of hindered thermal expansions and corresponding stress redistributions does not occur for reinforcing steel in tension. Therefore, the reinforcing steel is considered to act with its full strength, as written in equation (23a).
The proposed calculation scheme is illustrated in Figure 2, right hand side. The conditions are as follows:
Thermal stains and stresses can be neglected.
The concrete is represented with a constant temperature θM using the stress-strain curves of the Advanced Calculation Method.
The peak strain of the concrete |εc1,θ| is at least 3.5‰.
The stress-strain curves of the Advanced Calculation Method are used for the reinforcement.
The strength of the compressed reinforcement is reduced by ηs (θ), as given in equation (23b).
The proposed modification for the consideration of the effect of hindered thermal strains does only concern Step 6. Steps 1 to 5 are equal to the scheme proposed by Cyllok and Achenbach, as described in Section 3. The proposed function ηs (θ) for reduction of the compressed reinforcement is close to the approach by Cyllok and Achenbach, as displayed in Figure 8. Therefore, it can be supposed that the calibration will lead to comparable statistical key data, as given in Section 3. But reliable data can only be obtained by the recalculation of laboratory tests.
6. Worked example
The column displayed in Figure 9 has been tested at TU Braunschweig (Haß, 1987) as number 46 of the test series. The diameter of the stirrup is not given by Haß (1987). It is assumed to be 8 mm, in accordance with previous test series (Haß and Klingsch, 1980). The yield strength fyk of the reinforcement is considered with 526 MPa. The concrete strength βW,t has been measured with 200 mm cubes at the age of test. The equivalent strength fck is calculated by equation (24) with the factors proposed by Schnell and Loch (2009):
where k150 = strength of 150/200 mm cubes; kcyl = strength of cylinders/cubes; and kcure = strength of wet cured/dry cured concrete.
The moisture is considered with 3 per cent. The thermal conductivity is taken into account with its lower limit (EN 1992-1-2, 2004b). The temperature distribution of the cross-section is calculated with a finite difference scheme for every time step Δt = 1 min. The moment-curvature diagram of the cross-section is also calculated for each time step. For a given curvature, the strain at the centroid is varied until the inner forces NR,fi of the cross-section are equal to the applied axial force NE,fi to satisfy equilibrium.
The transfer matrix method with second-order effects (Petersen, 1982) is used for the determination of the state of strain. The column is divided in ten sections with constant stiffness, which is derived from the moment-curvature diagram. The failure of the column occurs when no equilibrium can be found.
The column failed in the laboratory at tf,exp = 50 min. The calculated time tf,cal for the Advanced Calculation Method is 45 min (−10 per cent) and 51 min (+2 per cent) for the extended Zone Method. The moment-curvature diagrams for both methods are displayed in Figure 10 for tf = 40 min and tf = 50 min. The curve for the acting moment ME,fi is plotted for reference. It is calculated with a nominal curvature, assuming a parabolic distribution:
where κ = curvature; NE,fi = acting axial force; e0 = eccentricity; and lcol = buckling length of column.
The shape of the moment-curvature curve of the extended Zone Method is comparable to the curve of the Advanced Calculation Method. In this example, the cross-section behavior of the Advanced Calculation Method is “softer” compared to the extended Zone Method. It is also obvious, that the line of the acting moment ME,fi and the curve of cross-section resistance MR,f do not have a clear intersection. This means that the results of calculations close to the ultimate limit state are dependent on the used calculation method and the chosen tolerance levels.
7. Conclusion
The basic ideas and assumptions of Hertz can be confirmed by the comparison with the results from explicit creep models. The manual calculation scheme can be extended toward a general method, suitable for the implementation in commercial design software. For the “hot design”, the material laws of the Advanced Calculation Method can be used, if the strength of the compressed reinforcement is reduced. One “rough” approach has been proposed by Cyllok and Achenbach (2009). This approach has been checked by the recalculation of laboratory tests (Cyllok and Achenbach, 2011).
This first approach is enhanced by introducing the factor ηs, which can be interpreted as a reduction of the area of reinforcement. In one worked example, taken from the tests carried out at TU Braunschweig, the results are close to the Advanced Calculation Method.
The recalculation of further laboratory tests is needed to calibrate the proposed extended Zone Method. The proof of equivalent structural safety, compared to the Advanced Calculation Method, can only be given by reliability methods.
Figures
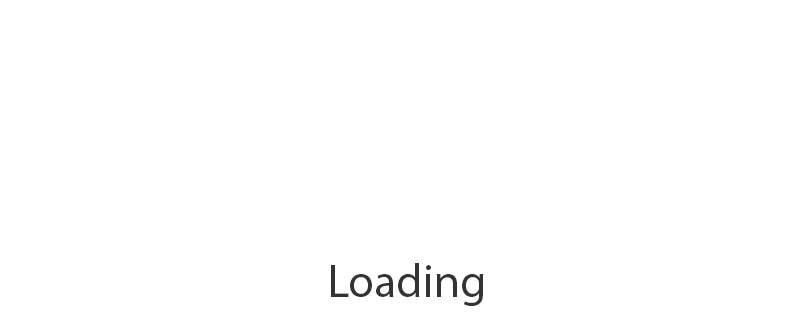
Figure 3.
Stress distribution in a wall (h = 12 cm, a = 1.8 cm, as,tot = 6 cm2/m, fck = 2 kN/cm2, fyk = 50 kN/cm2) after 30 min standard fire for EN 1992-1-2 (2004b) (EC2), Gernay and Franssen (GF), Anderberg and Thelandersson (AT), axial force −0.35 · NRd (left side), axial force −0.70 · NRd (right side)
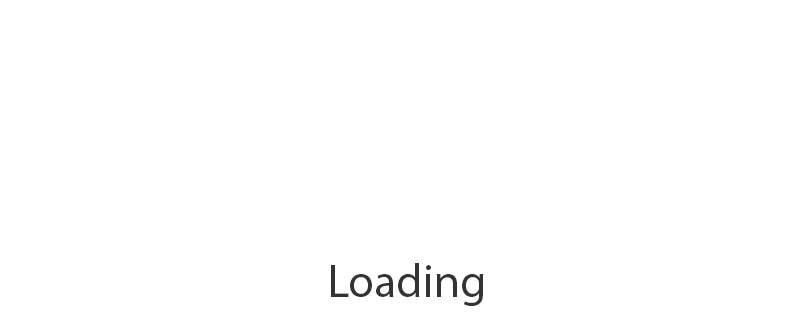
Figure 4.
Axial resistance NR,fi for compressed walls, results for EN 1992-1-2 (2004b) (EC2) on y-axis, results for the zone method (ZM), Gernay and Franssen (GF), Anderberg and Thelandersson (AT) on x-axis
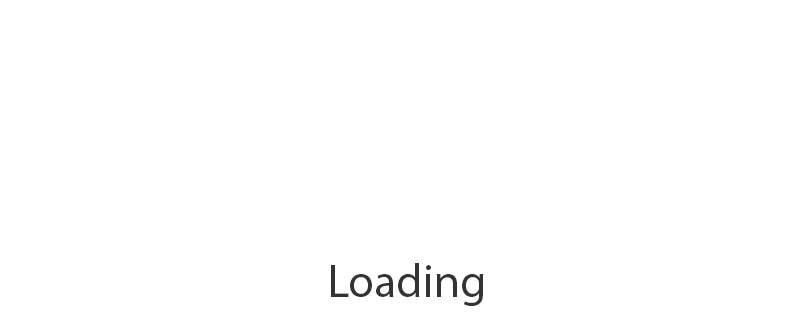
Figure 5.
Peak strains εc1,θ corresponding to fc,θ for EN 1992-1-2 (2004b) (EC2), the Zone Method (ZM), Gernay and Franssen (GF), Anderberg and Thelandersson (AT)
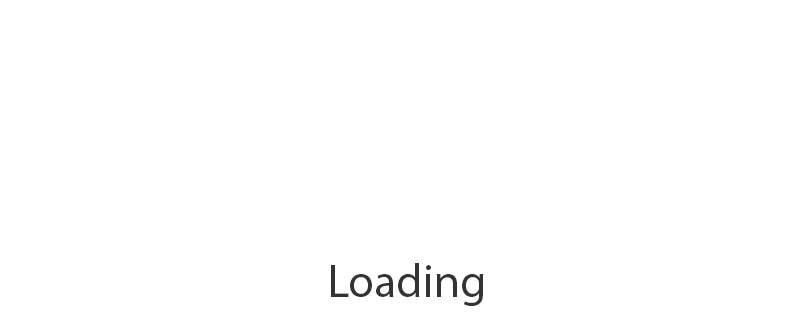
Figure 6.
kEc(θ) for EN 1992-1-2 (2004b) (EC2), the Zone Method (ZM), Gernay and Franssen (GF), Anderberg and Thelandersson (AT)
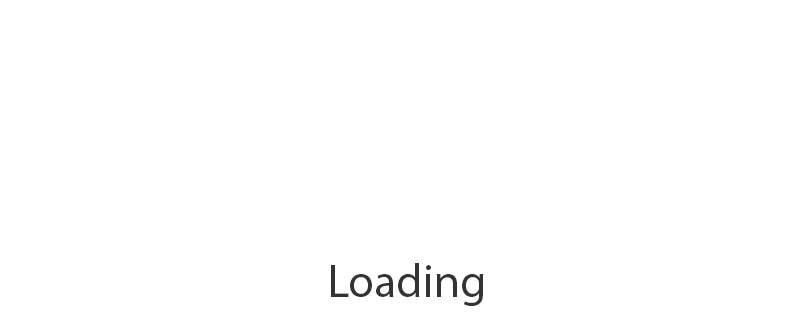
Figure 10.
Moment-curvature diagram for EN 1992-1-2 (2004b) (EC2), the Zone Method (ZM) after 40 min (left) and 50 min (right) standard fire, acting moment ME,fi(κ) (ME)
Parameters for compressed walls
h (cm) | a/h (−) | as,tot/h (%) | fck (MPa) | fyk (MPa) | tfire (min) |
---|---|---|---|---|---|
12 | 0.15 | 0.0 | 20 | 500 | 30, 60, 90 |
12 | 0.15 | 0.5 | 20 | 500 | 30, 60, 90 |
12 | 0.15 | 2.0 | 20 | 500 | 30, 60, 90 |
24 | 0.15 | 0.0 | 20 | 500 | 30, 60, 90 |
24 | 0.15 | 0.5 | 20 | 500 | 30, 60, 90 |
24 | 0.15 | 2.0 | 20 | 500 | 30, 60, 90 |
References
Anderberg, Y. and Thelandersson, S. (1976), Stress and Deformation Characteristics of Concrete at High Temperatures, 2, Experimental Investigation and Material Behavior Model, Lund Institute of Technology, Lund.
Cyllok, M. and Achenbach, M. (2009), “Anwendung der Zonenmethode für brandbeanspruchte Stahlbetonstützen”, Beton- und Stahlbetonbau, Vol. 12 No. 104, pp. 813-822.
Cyllok, M. and Achenbach, M. (2011), “Bemessung von Stahlbetonstützen im Brandfall: Absicherung der nicht-linearen Zonenmethode durch Laborversuche”, Beton- und Stahlbetonbau, Vol. 1 No. 106, pp. 39-44.
DIN EN 1992-1-2/NA (2010), Nationaler Anhang – National festgelegte Parameter – Eurocode 2: Bemessung und Konstruktion von Stahlbeton- und Spannbetontragwerken – Teil 1-2: Allgemeine Regeln – Tragwerksbemessung für den Brandfall, DIN, Berlin.
EN 1992-1-1 (2004a), Eurocode 2: Design of Concrete Structures – Part 1-1: General Rules and Rules for Buildings, CEN, Brussels.
EN 1992-1-2 (2004b), Eurocode 2: Design of Concrete Structures – Part 1-2: General Rules – Structural Fire Design, CEN, Brussels.
ENV 1992-1-2 (1995), Eurocode 2 - Design of Concrete Structures – Part 1-2: General Rules – Structural Fire Design, CEN, Brussels.
Gelien, M. (2011), “Ein Beitrag zur Bemessung von Stahlbetonstützen im Brandfall”, Dissertation, Bergische Universität Wuppertal.
Gernay, T. and Franssen, J.-M. (2012), “A formulation of the Eurocode 2 concrete model at elevated temperature that includes an explicit term for transient creep”, Fire Safety Journal, Vol. 51, pp. 1-9.
Haß, R. (1986), “Zur praxisgerechten branschutztechnischen Beurteilung von Stützen aus Stahl und Beton”, Dissertation, TU Braunschweig.
Haß, R. (1987), “Brandversuche an Stahlbeton- und Verbundstützen”, in Sonderforschungsbereich 148, Brandverhalten von Bauteilen, Arbeitsbericht 1984-1986, Teil I/A, Braunschweig, pp. 80-99.
Haß, R. and Klingsch, W. (1980), “Parameteruntersuchungen zum Brandverhalten von Stahlbetonstützen – Versuche und theoretische Begleitung”, in Sonderforschungsbereich 148, Brandverhalten von Bauteilen, Arbeitsbericht 1978-1980, Teil I, Braunschweig, pp. 91-112.
Hertz, K. (1985), Analyses of Prestressed Concrete Structures Exposed to Fire, Technical University of Denmark, Lyngby.
Holmberg, S. and Anderberg, Y. (1993), Concrete Structures and Fire: Computer Simulations and Design Method for Fire Exposed Concrete Structures, Fire Safety Design, Lund.
Petersen, C.H. (1982), Statik und Stabilität der Baukonstruktionen, 2nd Auflage, Vieweg, Braunschweig/Wiesbaden.
Richter, E. and Zehfuß, J. (1999), “Erläuterungen und Anwendungshilfen für die brandschutztechnische Bemessung mit Eurocode 2 Teil 1-2”, in Gleichwertigkeit von Brandschutznachweisen nach Eurocode und DIN 4102, Tl. 4, Fraunhofer IRB Verlag, Stuttgart.
Schnell, J. and Loch, M. (2009), “Umrechnung historischer Baustoffkennwerte auf charakteristische Werte”, Der Prüfingenieur, Vol. 34, pp. 50-61.
Zilch, K., Müller, A. and Reitmayer, C. (2010), “Erweiterte Zonenmethode zur brandschutztechnischen Bemessung von Stahlbetonstützen”, Der Bauingenieur, Vol. 6 No. 85, pp. 282-287.