Abstract
Purpose
The overall objective of this study is envisaged to provide decision makers with actionable insights and access to multi-risk maps for the most in-danger stave churches (SCs) among the existing 28 churches at high spatial resolution to better understand, reduce and mitigate single- and multi-risk. In addition, the present contribution aims to provide decision makers with some information to face the exacerbation of the risk caused by the expected climate change.
Design/methodology/approach
Material and data collection started with the consultation of the available literature related to: (1) SCs' conservation status, (2) available methodologies suitable in multi-hazard approach and (3) vulnerability leading indicators to consider when dealing with the impact of natural hazards specifically on immovable cultural heritage.
Findings
The paper contributes to a better understanding of place-based vulnerability with local mapping dimension also considering future threats posed by climate change. The results highlight the danger at which the SCs of Røldal, in case of floods, and of Ringebu, Torpo and Øye, in case of landslide, may face and stress the urgency of increasing awareness and preparedness on these potential hazards.
Originality/value
The contribution for the first time aims to homogeneously collect and report all together existing spread information on architectural features, conservation status and geographical attributes for the whole group of SCs by accompanying this information with as much as possible complete 2D sections collection from existing drawings and novel 3D drawn sketches created for this contribution. Then the paper contributes to a better understanding of place-based vulnerability with local mapping dimension also considering future threats posed by climate change. Then it highlights the danger of floods and landslides at which the 28 SCs are subjected. Finally it reports how these risks will change under the ongoing impact of climate change.
Keywords
Citation
Bertolin, C. and Sesana, E. (2024), "Natural hazards affecting cultural heritage: assessment of flood and landslide risk for the 28 existing Norwegian stave churches", International Journal of Building Pathology and Adaptation, Vol. 42 No. 1, pp. 48-91. https://doi.org/10.1108/IJBPA-03-2022-0042
Publisher
:Emerald Publishing Limited
Copyright © 2023, Chiara Bertolin and Elena Sesana
License
Published by Emerald Publishing Limited. This article is published under the Creative Commons Attribution (CC BY 4.0) licence. Anyone may reproduce, distribute, translate and create derivative works of this article (for both commercial and non-commercial purposes), subject to full attribution to the original publication and authors. The full terms of this licence may be seen at http://creativecommons.org/licences/by/4.0/legalcode
1. Introduction
The Sendai framework for disaster risk reduction (DRR) 2015–2030 (United Nations Office for Disaster Risk Reduction, 2015) marked a clear shift respect to its predecessor, the Hyogo Framework for Action (HFA) 2005–2015 (United Nations Office for DRR, 2005), from managing the impact of disasters to managing and reducing risks that lead to disasters. The concept of DRR as described in HFA reflects a stronger focus on risk preparedness and prevention, as opposed to the emphasis on response and recovery adopted by the Yokohama strategy during the previous decades (IDNDR, 1994). Science, technology and research play a leading role in improving risk awareness and governance, so that an effective move from reaction to prevention may become possible. The Sendai framework identifies the analysis and ongoing monitoring as one of the main objectives to put in place; multi-hazard early warning systems and the strengthening of disaster preparedness is essential to reduce human and material losses. According to UNISDR, 2015, the multi-hazard concept refers to both the selection of multiple hazards an area is facing and the context in which hazards may occur simultaneously as cascade or cumulative events over time, considering potential interconnected effects. This requires access to existing data and/or data collection to evaluate risk and thus inform decision makers (e.g. risk management experts, advisors, insurance, critical infrastructure operators, owners and cultural heritage managers) about current and future threats at local scale. Among natural hazards, flood is defined as a temporary covering by water and sediments over land outside the watercourse. It includes rivers flooding in flood plains, lakes flooding and sea water flooding.
Flooding may be caused by many factors such as intense rainfall, ice jam, melted snow over an area already saturated with previous precipitation and/or lakes, riverbeds and soil and vegetation that cannot absorb the excess of water that therefore travel over land as surface runoff. Strong winds over water or unusual high tides may be another cause of flooding, especially in coastal areas. Even in the case of light rain, the coast may be overflooded due to an overlapping result of strong winds and high tides. An impact resulting from a sudden flood might be a landslide defined as a geological and climatologically phenomenon that triggers mass movements i.e. detachment and transport of soil, rocky material (e.g. rockslides or falls) or liquid material (e.g. flows) slope down. Three main factors which influence landslides occurrence are the type of soil (e.g. fine or coarse grained and cohesion level), the slope and the soil water content. The latter can be modified naturally by rapid snowmelt, intense rainfall and storm waves, or anthropically by human activities as poor land management and forestry practices, land use change (e.g. deforestation, conversion of land), construction activities and soil sealing. During a landslide event, the loose material, along with the overlying soils, may move either slowly or rapidly (debris flows).
These two types of hazards can cause a rapid shift in the degradation conditions of historic buildings, monuments and artefacts (Sesana et al., 2021; Leissner et al., 2015; Brimblecombe et al., 2011; Sabbioni et al., 2010). Climate change can alter the intensity and frequency of these hazards modifying their location and occurrence. In Norway, the climate is expected to change in terms of snow cover, temperature increase (felt mostly in the winter season in the Northern regions), and average annual precipitation. Increases in precipitation as well as the reduced capacity of water basins to convey with sudden or high-water flow, raise the risk of flooding events. These factors might accelerate rot in absorbent building materials, such as wood (Grøntoft, 2019), as well as biological degradation by fungi, mould and insects (Haugen and Mattsson, 2011). The annual runoff from the Norwegian mainland is estimated to increase, but less than annual precipitation since evapotranspiration also will increase. The increase in precipitation, especially when extreme, will lead to a rise in flooding events. Future changes in flood magnitudes have been analyzed for 115 rivers in Norway (Lawrence, 2016). Although the direction of change is the same in all the studies, the results show that the magnitude of change strongly depends on the considered emission scenario. It is expected that the general pattern of change will vary across regions, with coastal flooding influenced also by the rise of the sea level along the coasts, and then that rain flood magnitudes will increase while snowmelt flood magnitudes will decrease. The increases in temperature will lead to events of summer droughts and to the melting of glacial areas (Lawrence and Hisdal, 2011; Wilson et al., 2011; NVE, 2016b; Hanssen-Bauer et al., 2018). Concerning landslides, a modification in weather conditions may impact earth slides that mainly occurred in steep terrain as flood slides, rockslides triggered by heavy rain and/or snowmelt and smaller rockfalls triggered by freezing and thawing (Hanssen-Bauer et al., 2018). Therefore, it is expected that climate change will affect the landslide frequency for wet snow avalanches and earth, flood and slush slides.
1.1 Governance aspects of floods and landslides: European directives and the Norwegian state legislation
As reported by the European Union (EU) Environment Agency in 2017 (EEA, 2017a, b) over the 1980–2015 period, Europe – due to catastrophic floods – was affected by €164 billion in economic losses (Kleinen and Petschel-Held, 2007). To reduce human and economic losses as well as irreversible damages on landscape and cultural heritage, it is since autumn 2002, after the devastating flood that affected Central Europe that the Water Directors of the EU, Norway, and other nonEU countries decided to jointly act in the field of flood protection. Besides the HFA (UNISDR, 2005) and the Sendai Framework for DRR (UNISDR, 2015), the sign of their commitment has been the creation of the EU Flood Directive (FD) (Directive 2007/60/EC) and the EU Inspire Directive (European Directive 2007/2/EC). These directives have strongly influenced the development of national strategies and legislation on the assessment and management of flood risks and on the establishment of spatial information infrastructure (publicly available) both in EU countries (obliged to implement them) and nonEU countries (not obliged). Following the commitment of the EU FD, the member countries must produce preliminary risk assessment (2009–2018, FD 1st and 2nd cycle), conduct flood mapping (2013–2019, FD 1st and 2nd cycle) and implement flood risk management plan (2015–2021, FD 1st cycle). Then the whole cycle needs to be revised every six years because the flood risk is not constant over time.
Landslides occur in many different geological and environmental settings across Europe. Rockfalls, rockslides, rock avalanches and debris flows dominate in the Alps and steep slopes. In continental Europe, intense and long-lasting rainfall is frequent triggers of landslides. In Norway, although the slopes over 30° steepness are less than 7%, circa one-third of the total land area is covered by mountains, e.g. with the Scandinavian chain that is crossing the country from south to north and is dividing it into western and eastern parts. In such a territory, large areas are exposed to several types of mass movements: snow avalanches during wintertime that affect large parts of western and northern Norway, slush flows during early winter and spring, rock slides and debris slides which occur during the whole year and are most common in western Norway and in the Troms area.
In Europe, landslides are one of the soil threats recognized by the EU's thematic strategy for soil protection that proposes soil framework actions and tools for the protection and sustainable use of soils to be implemented by 2030, in synergy with other European Green Deal policies. The adoption of this ambitious strategy is very recent (November 2021), it highlights as the EU is suffering by different levels of land degradation (e.g. erosion, landslides, salinization, droughts and desertification) and that a wide range of consequences, exacerbated by natural hazards, need to be faced in EU’s member countries (European Agency’s 2020 State of the Environment Report, Special IPPC report on Climate Change and Land and IPBES Assessment Report on Land Degradation and Restoration). The strategy also explains how a proper protection and management of soil is crucial for climate change mitigation and adaptation, and for cultural heritage protection; indeed, the soil is part of cultural natural landscape and serves as platform for cultural heritage assets. Finally, soil must be considered as a nonrenewable resource, which is shrinking, that is continuously under pressure from urbanization and infrastructure. Overall, the strategy requires identifying landslide risk areas in the EU, to set of risk and to implement programs of risk management measures.
Examples of EU guidelines in flood mapping’s best practice have been reported in the European Flood Atlas (Handbook on flood mapping in Europe, De Moel et al., 2009). Flood and landslide mapping are useful in displaying the effect of geohazards (e.g. stagnant or flowing water outside the bed or the shoreline, salinization, sediments and erosion). Hazard maps and risk maps are the most developed in Europe. The former contains information about probability and/or magnitude, for example highlighting areas flooded or covered by debris flow accordingly to different (historic and future potential) probabilities or illustrating the intensity and magnitude of hazard at a selected scale. The latter indicate potential adverse consequences caused by floods and landslides under different probability or return periods. However, very few countries in Europe have produced risk maps under the FD because, being the risk defined as probability of an event multiplied by its consequences for their realization, they require the analysis of the magnitude and probability (hazards), the damage potential (exposure) and the way the events are dealt with (coping capacity) each step in the analysis connected to an assessment criterion. These nation/basin wide maps are mostly developed by centralized governmental organization, trans-national river basin authorities and by insurance industry and primarily used for emergency planning, spatial planning and awareness rising. In Norway, following the EU FD, the most common produced hazard maps for both floods and landslides are the extent and danger maps. They are made by the Norwegian Water Resources and Energy Directorate’s (NVE) and report depicted with colors the farthest hazard extent under the most extreme return period of 1,000 years containing the less extreme ones (200-years and 20-years, respectively). These maps are therefore considering the potential maximum risk (i.e. worst case scenario) leading to maximum negative consequences.
As reported by Kapović Solomun et al., 2022, since 2007 nonEU countries have started transposing the EU FD into own national legislation, sometimes with weak mutual cooperation and synchronization results. This is due to multiple causes such as issues with decentralized type of governance, economic status of the country (e.g. under development), wide number of competent authorities that manage flood and landslide risk, complex administration, lack of harmonized strategy and investment plans for water and soil management, and need of building new, large and expensive structural defenses.
In Norway (a non-EU country), within the framework of climate adaptation with implications for natural hazards, the Norwegian Environment Agency supports the Ministry of Climate and Environment in working to increase DRR knowledge base to prevent and limit consequences of natural hazards. The actors involved are the Directorate for Civil Protection (DSB) that supports the Ministry of Justice and Public security in coordinating civil protection and emergency planning efforts in Norway, and the Ministry of Petroleum and Energy that together with the NVE has the responsibility for managing the risk of floods and landslides at the national level. NVE has been given a central role in the implementation of EU FD in Norway, as it is the executive authority producing mapping programs and warnings at national level. Although Norway has a decentralized land use planning system, giving local authorities the juridical role of main land use authority, NVE supports municipalities through advice in spatial planning and/or in planning for construction of structural protection measures. All the concerns that are described in the Norwegian Planning and Building Act (2008), the Cultural Heritage Act, the Public Health Act and the Biodiversity Act are met in the land use planning processes at local level. On this legislative background, Norway is implementing the Water Framework Directive under a specific timetable pursuant to the Agreement on the European Economic Area (EEA). The preliminary risk assessment in the first cycle was completed by Norway in 2011 (NVE, 2011). During the 2012–2015 period, NVE, the Norwegian Public Roads Administration and the Norwegian National Rail Administration worked together in the project “Natural hazards, infrastructure, flood and landslides” (NIFS, www.naturfare.no) to contribute to a better understanding of roles, skills and competences, as well as a long-term planning for coordinating natural hazards assessments and a safer and prompter risk management (NVE, 2016b).
A national strategy dealing with floods, landslides and avalanches was issued by the Norwegian Government in 2012 in the White Paper “How to live with the hazards”. As occurred with the Norwegian flood risk management, the NVE is the operational authority responsible for landslide risk management at the national level, together with the DSB having a leading role in emergency preparedness and response. The municipalities are responsible for local civil protection, DRR, and for taking into account risk of landslides in land use planning and building construction. Partners for landslides inventory DBs are National Road Authority, National Railway Authority (e.g. events with impact on transport infrastructures), NGU (e.g. DB on historic events), and the Norwegian Geotechnical Institute (NGI). All these landslides DBs are hosted at NVE since January 2014 in compliance with EU directives. The NVE portal is used in this contribution.
To date, the gaps and challenges that have been recognized are the following:
Need for clarification at regional/local level of crisis management roles
Need of harmonization of risk levels criteria and their acceptance
Need of standardization of risk maps, shared data, terms and information in common national databases
Need of improving land use planning on long-term, embedding impact of future climate change scenarios
Need of assessing risk by water (and/or drainage paths) over whole catchment areas considering the greater consequences of hazards downstream
Need of developing an overall methodology for assessing socio-cultural-economic consequences of hazards that cause damage
Need of planning structural (and nonstructural) measures that should be more robust to withstand climate change impacts
Further research is recommended in this direction in order to face the plans for the 2022–2027 cycle of Norway’s formal obligations.
1.2 State of the art of cultural heritage risk under threats by floods and landslides
Over the last 12 years, research addressing flood risk assessment, specifically on churches, has been focused on the study of hazard, vulnerability and risk over scenarios with different return periods ranging from 10 to 1,000 years (Moreno et al., 2022; Figueiredo et al., 2021; Loreto et al., 2021; Previtali et al., 2018, MICHE Project), on the reconstruction of past periods using mapping or datasets (Ortiz et al., 2016) and on the comparison of years in the past when extreme events occurred (Previtali et al., 2018; Cuca, 2020). Similarly, this has been done for landslides, although for this hazard is more common to have building structural monitoring performed in continuous or sporadic geotechnical or laser scanner surveys at support of a risk assessment over an extended time (Cambiaggi and Berardi, 2019; Indirli et al., 2011; Cigna et al., 2013).
The literature review shows clearly that the research community is well aligned in terms of research-driven policy framework, as all the works related to churches affected by flooding quote the EU FD, several the Sendai DRR framework, while few the INSPIRE directive (Moreno et al., 2022; Cuca, 2020). Recent EU and National projects who have driven research on Flood risk assessment and management are: the EU-FP6 Cultural Heritage Protection against Flooding (CHEF), the Risk and Vulnerability in Cultural Heritage (RIVUPH) project funded by Andalusian Government (Ortiz et al., 2016), the EU Risk Indicators for the Analysis of Cultural heritage under Threat project (RIACT) (Figueiredo et al., 2021), the Italian MICHE – mitigating the impact of natural hazards on cultural heritage sites, structures and artefacts project for the city of Florence, the NOAH web application program with historical/real time natural hazards and weather events in the Philippines (Loreto et al., 2021) and the National Flood Insurance program for the Federal Emergency Management Agency (FEMA) high-risk ranking zone in USA (Paille et al., 2016). Interestingly, few works quote the involvement of local municipalities as driving factors in planning research (Previtali et al., 2018 and Indirli et al., 2011 in case of landslide hazard). The selection of the churches as case studies is often based on existing information or maps that highlight their high exposure e.g. flood or landslide susceptibility maps (Cambiaggi and Berardi, 2019; Pascale et al., 2013), created by overlapping cultural heritage assets' location or based on knowledge of past hazards (Previtali et al., 2018; Cuca, 2020; Ilieș et al., 2020). Countries where the research has been conducted might indicate zones more prone to disasters: Portugal, Spain and Italy in the Mediterranean area, the Louisiana in the US and, for multi-hazards, the Philippines. In Europe, Italy is the country with a higher number of studies on regions (e.g. Liguria) at high risk of hydrogeological instability with an expected return period of dangerous floods of circa 50 years (Previtali et al., 2018; Cuca, 2020; Cambiaggi and Berardi, 2019; Cigna et al., 2013; Pascale et al., 2013). Unless the number of studies, research highlights the difficulties of building structural measures for DRR, especially in a region like Liguria which is confined between the mountainous territory and the coast, with little space available, or appropriate, for the realization of measure to reduce flooding risk, i.e. dams. Paille et al. (2016) has reported as in England, in the Netherlands and in Germany, strong flood mapping tools drive planning decisions, but – although these tools still run – the risk is remaining considerable. Paille et al. examine the Louisiana (USA) context under which coastal parishes face flood risk management through the adoption of a Community Rating System (CRS), which encourages in implementing structural and nonstructural flood RR measures for having a discount on flood insurance rate. CRS is based on score evaluation and has a range of activities as: public information, mapping and regulations, flood damage reduction and flood preparedness. Although CRS program still sees resistance between central and local governments, the researchers have found that “non-structural” methods, such as those measured by the CRS rating, were more than twice as effective as “structural” measures in DRR. Looking at the number of churches investigated in each study, interestingly, for flooding risk assessment often from 7 to 30 churches locations are compared (Ortiz et al., 2016; Moreno et al., 2022; Loreto et al., 2021). For landslide, only Pascale et al. in 2013 assess a risk prone area with more than one church, while all the other reviewed studies remain focused on a single church.
The methodologies adopted in literature which study flooding and dampness as well as landslide and mass movement in historic city centers are multiple. GIS software is often used to assess hazards through mapping, and to create digital archives with information and scenarios of specific and multiple risk to assist future urban planning (Ortiz et al., 2016; Cambiaggi and Berardi, 2019; Indirli et al., 2011; Ilieș et al., 2020). GIS is integrated with other tools such as Delphi (Ortiz et al., 2016) i.e. a consultation process surveying a multidisciplinary group of experts to gain weighted factors, or as the frequency ratio method to create susceptibility index (Ilieș et al., 2020). Previtali et al. (2018) recognized the need of a further step in this proposed monitoring methodology i.e. to include in the GIS system, remote sensing data as data multilayers. The earth observation technologies (e.g. Copernicus Sentinel-2, ERS1/2, ENVISAT and RADARSAT1 data) have already been used in monitoring and assessing risk on two churches in Italy (Cuca, 2020; Cigna et al., 2013) for both performing rapid mapping with an immediate response, and for detecting changes over prolonged periods of time after the event. This helps experts in documenting losses of valuable material or in gaining better insights on the induced damages. More recently, as reported by Romao and Bertolin in 2022, these topics started to involve the combination of machine learning (ML) techniques with remote-sensed data for identifying the threat level of multi-hazards for early warning systems development (Moreno et al., 2022) or for evaluating and mapping landslide susceptibility. The latter was done by Pascale et al. (2013) using an artificial neural network (ANN) that requires the definition of appropriate thematic layers to parameterize the area under study. Moreno et al. (2022) show the effectiveness of Art-risk 3.0, a ML fuzzy logic platform, in identifying the threat level. In addition, they assess the degree of vulnerability using 14 variables assessed through bibliographic search and review of photographic images, and a functionality index interrelating hazard and vulnerability to determine the useful life of historical churches in Spain. Concerning data driven methodologies, the identified gaps in knowledge are related to the systematic and homogeneous use of citizen science to update flood risk management data. In fact, both ML and citizen science through public engagement could improve the capability in detecting buildings pathologies through photographs. From the review appears clear as the research addressing risk assessment has been largely dedicated to mapping under different hazard scenarios as main methodological approach. This is also the case of the MICHE project, where mapping outcomes are the result of risk assessment presented for cultural buildings and for artworks in terms of annual average loss. Despite this agreement, research addressing the vulnerability modeling of cultural heritage is still limited with some studies focused on issues related to the exposure of cultural heritage to flood as that of Figueiredo et al. (2021) that developed a specific depth-damage vulnerability function for cultural heritage buildings that were component-based, including building envelope and its content. On this aspect the recognized gaps in knowledge are the lack of existing approaches in the literature to achieve quantifiable flood and landslide risk for prioritization, the application of these tools to other types of cultural heritage buildings and the analysis of uncertainty that stems from translating the susceptibility and value of asset components into indices.
Similarly, very few studies exist on the consequences of damages on cultural heritage. A good example here is the MICHE project which maps the loss of value, while other studies (Figueiredo et al., 2021; Loreto et al., 2021; Previtali et al., 2018) report as it is of fundamental importance (although not always done) to conduct repetitive surveys (before/after events, or repetitive over time), collect data (e.g. on age, materials, histories of degradation, alteration, improvements), assess hazard susceptivity on material and/or technique to be able to convert all in relative value indices assessment. Other recognized limitations are as follows: the lack of data and information on foundations of churches that interact with the subsoil during groundwater rise (Ortiz et al., 2016), and the need of associating failure characteristics (pathologies) in churches to hazard, vulnerability and functional indices, as these direct connections may support a sustainable use of budget available for preventive conservation (Moreno et al., 2022).
Finally, as stated by Previtali et al. (2018), what in the past was defined as exceptional, nowadays has to be considered ordinary because of the higher vulnerability of the territories and the hazards exacerbated by climate change. This of course changes our perspective on the classification of the exceptional catastrophic events and highlights the need of effectively working to update preparedness and management plans globally.
1.3 The Norwegian stave churches as case study and the aim of this paper
To better cope with these recognized needs and uncertain future, with the increasing frequency and magnitude of flood and landslide disasters as well as with their DRR, it is important to take local actions for effective risk management. This is the case of urban area and/or cultural landscape, where the impact of natural hazard can be very high in terms of human and/or heritage loss. In Norway, such highly valuable cultural landscape is represented by the 28 locations that still preserve the last existing medieval stave churches (SCs). Given the potential threats that natural hazards might pose for the Norwegian “Stavkirke”, this research presents an assessment of the potential multi-hazard vulnerability and risk assessment methodology for flooding and earth landslide events. A Norwegian wooden medieval SC, also called “Stavkirke”, is a building with a frame structure made of horizontal and vertical wooden load-bearing elements called staves or posts, resting on stone foundations. Only 28 SCs, on about two thousand, survived till our ages in Norway and one of them, the Urnes SC is listed as a UNESCO World Heritage Site [1] (Bakken et al., 2016; Lehne et al., 2019). Nowadays the SCs can be found in only three countries in Europe: Norway, Sweden and the United Kingdom; however, similar structures where common in all the northern European countries in the middle ages (Lehne et al., 2019). In Norway, the SCs can be found in the central and southern regions of the country, most of them are located near waterways or in hilly and mountainous landscapes and can therefore be exposed to natural hazards. Sudden events, such as flooding and landslide, pose a threat to the preservation of cultural heritage, especially when coupled with climate change; the rising of sea level, for instance, combined with tides and storm surges can inundate coasts, also causing its erosion (Colette, 2007; UNESCO, 2008; Sabbioni et al., 2010; Brimblecombe et al., 2011; Leissner et al., 2015; ICOMOS, 2019; Sesana et al., 2021). Such risks need to be taken into consideration to plan the preventive preservation and preparedness plan of the few remaining SCs; indeed, the SCs are important given their inherent memory, history and their significance for the associated communities (Lehne et al., 2019).
In 2001 the Norwegian Directorate for Cultural Heritage started an initiative for the preservation of the SCs which were found to be in poor conditions. Within the Stave Church Preservation Program (SCPP) [2] (Bakken et al., 2016) the 28 churches were repaired by, for example, building new foundations, restoring from rot damage and repairing the slate roofs. Even though the strong efforts made by the Norwegian Directorate, with the conditions of the churches that are checked each year and the owners that can have economic support through grants for maintenance work, the churches might experience adverse consequences under the action of natural hazards. For the SCs, the cultural environment authorities have already requested to the Norwegian Institute for Cultural Heritage Research (NIKU) – on commission from the Directorate for Cultural Heritage – to conduct a survey for detecting which of them are particularly vulnerable to climate change. The outcomes recognized as water poses the greatest threat linked to increased biodegradation (66% of SCs with damages as mould, fungus, rot caused by high wood moisture content), while SCs located in hazard prone areas (ca 33%) will be likely at risk of avalanches, landslides or rock falls. The open challenges and gaps in knowledge are related to the implementation of adaptation measures to the whole group of the 28 SCs, in particular to divert water from roofs into permeable ground and ensuring adequate drainage.
Since 2020, NIKU, the Norwegian Association for Church Employers (KA) and external advisers, in collaboration with the Directorate for Cultural Heritage are working to generate concrete knowledge about the risk of flooding, and landslides to wooden and stone churches protected by the cultural heritage act to inform church owners and municipality about risks, responsibilities and appropriate measures they can take to work effectively with authorities in protecting the most vulnerable churches and thus reducing risks.
The overall objective of this paper is to contribute to this main goal, understanding the danger at which the SCs are posed, and to inform decision makers by giving them access to independent multi-hazard, high spatial resolution, risk maps, specifically for the SCs at most danger among the existing 28. This paper will deal with independent multi-hazards category where single hazards are just overlayed and treated as independent phenomena. Although such approach to multi-hazard can potentially underestimate the risk leading to not always adequate DRR measures. However, it is a well-established and understood procedure that allows to better perceive, reduce and mitigate single and independent multi-hazard risks fixing the right priority scores. In addition, the present contribution aims to provide decision makers with some information to face the exacerbation of the risk caused by the expected climate change. The specific outcomes are as follows.
Review of geographical, architectural, cultural and historical information for the 28 SCs and their homogenization
Existing flood and landslide risk in proximity of the 28 SCs using an index-based approach (IBA) to identify the top five SCs at risk of floods and landslide
Use of the NVE danger maps to highlight the risks
Suggestion on how to use and improve these maps
2. Material and methods
Material and data collection started with the consultation of the available literature related to (1) SCs conservation status (e.g. Olstad, 2016; Anker, 2016; Olstad and Solberg, 1998; Wedvik, 2008, 2009; Solberg et al., 2007; Olstad and Ørnhøi, 2014; Bakken et al., 2016; Lehne et al., 2019), (2) available methodologies suitable in multi-hazard approach; specifically, an IBA built on previous work by Miranda and Ferreira (2019), Ferreira and Santos (2020) (see Section 2.1) was developed and applicated to the SCs' case studies (see Section 2.2).
The literature search was conducted in English on the “Scopus” database using the following keywords with the Boolean operator AND: “church”, “risk”, “flood” (for flood risk a total of 26 papers fitted the search, 13 were read in full) and “church”, “risk”, “landslide (for landslide risk a total of 11 papers were identified, 9 read in full). In addition, further articles, grey literature and information on the SCs were retrieved in both English and Norwegian language on the NVE website (https://www.nve.no/english/) as well as on reports developed from national institutions such as the Norwegian Directorate (https://www.riksantikvaren.no/en/).
During the process of data collection and literature review, plans and 3D images for the 28 churches were searched. Plan schemes were found for only 21 out of 28 churches in existing publications and/or database (https://kulturminnebilder.ra.no/fotoweb/archives/5002-Tegninger/). The latter, is a repository developed by the Norwegian Directorate which make publicly available information, documents and images about Norwegian Heritage. For this specific search, the keyword “Stavkirker” was used to identify 850 images contained in the repository of which only 25 served as reference for the plan images reproduced in this contribution; the full references and images credits references are reported in Appendix 1. Starting from these diversified schemes, new plans were homogeneously reproduced with the use of the Autocad® Software; they do not correspond to the geometrical surveys of the churches in their current form as the images of reference correspond to different time periods (1800–1900, see Appendix 1). The 3D schemes were also digitally hand-drawn with a graphic tablet, the references for the church images derived from the pictures available in the literature (e.g. Bakken et al., 2016) and on the Google Images website. Once the geographical conditions and architectural features of the 28 “Stavkirke” as well as their conservation status (e.g. refurbished, rebuilt and relocated) were defined, the risk of natural hazards, i.e. flooding and landslides events, for the locations where the churches are placed was investigated. The whole structure of the paper with the methodological approach adopted is illustrated in Figure 1.
2.1 The index-based approach (IBA)
As stated in section 1,2, when working with immovable cultural heritage, the gap in knowledge stays in the vulnerability assessment. Analytical tools and risk ranking schemes are used to distinguish levels of vulnerability. One of the ranking methodologies used in heritage field to assess vulnerability is known as the IBA method that is a semi-quantitative approach. The strength of this tool is its user-friendly procedure, its application in previous scientific works devoted to the analysis of risk of flood for churches, and its easiness in defining components of risk in precise and predominantly quantitative way.
The major IBA weakness stay in the semi-quantitative determination of vulnerability and risk that uses a scale of units that is not calibrated but that is delivered in form of range of frequency and/or consequences levels. For this reason, the final risk can be assessed in a relative way with a remaining of subjective components that – due to the lack of calibration studies – cannot be avoided. This means that IBA allows depicting scenarios that rank vulnerability from one scenario to another or from a single SC and its location to a group of similar SCs and or comparable locations. Two types of inconsistencies may derive from applying IBA to the SCs case study: a first type which may place in the same risk level scenario two or more SCs – that although being directly comparable – may have (in reality) different risk grades; and a second type of inconsistency which may place in the same risk level scenario SCs that may be not completely comparable e.g. due to their different climate zones. Finally, a weakness stays also in the difference between the level of accuracy obtained for the hazard related information (often quantitative), and those obtained for risk consequences (often qualitative) that contain implicit quantitative values not easy recognizable. In this contribution an improved IBA was built on the previous work by Miranda and Ferreira (2019), Ferreira and Santos (2020) and is here reported in Figure 2.
The IBA is composed of five original indicators for the sensitivity component:
Material (physical dimension) is classified according to general material classes (as illustrated in Figure 2). In the case of structures with multiple materials, it is desirable to select the weakest.
Condition of the building envelope (physical dimension) i.e. level of maintenance and preservation of the building envelope.
Number of storey (physical dimension), i.e. vertical regularity of the building and the type of floors.
Age of the historic building (cultural dimension), i.e. how the historic value is affected by the hazard, assuming that older constructions are more likely to have more historic value.
Heritage status (cultural dimension) i.e. building significance at local, national or international level.
And an updated indicator added in this work:
Heritage content (cultural dimension) i.e. susceptibility of indoor immovable and movable heritage. This cultural dimension has been added, following the main scope of the FD i.e. monitoring and forecasting consequences of floods for a better management of flood risk and for developing effective measures to reducing the effects of geohazards. In addition, the heritage content susceptivity was also integrated in implemented methodological approach used by the EU project climate for culture (2009–2014) to conduct damage risk assessment, economic impact, and mitigation strategies in time of climate change (Leissner et al., 2015).
For what concern exposure component, the original IBA had a single indicator:
Wall orientation and openings i.e. building orientation facing the direction of the hazard in the danger area and the dimensions of openings in the exposed wall.
Three extra exposure components (topographic) indicators were added as follows:
Altitude used to understand the local relief as landslide has a higher tendency to occur at higher altitude (Youssef et al., 2015).
Slope i.e. steepness of the terrain between cultural heritage and the hazard Area (HA), is one of the factors increasing or decreasing exposure of a cultural heritage resource to a floods or landslides hazard and is widely used in susceptibility mapping (Park et al., 2013).
Distance of the SC from the HA.
A multi-hazard risk assessment constitutes an essential tool to designate further analyses, strengthen resilience or plan DRR interventions (Figueiredo et al., 2020, 2021). This simplified method allows, with a relatively small amount of information, to take into consideration the tangible/intangible value of CH (Sevieri et al., 2020). Following the procedure reported in D`Ayala et al. (2006), Julia and Ferreira (2021) and in Paupério et al. (2012), the single and independent multi-hazard risk assessment is conducted over the cultural heritage sites’ locations (with j ranging from 1 to n. numbers of heritage sites) using equations (1) and (2) (single approach) and 3 (independent multi-hazard approach):
These equations are linear aggregations of indicators (Ii, with i = 1 to 10 referred to Figure 2) multiplied by their weights for flood and landslide Wflood,I and Wlandslide,I, respectively. The weights associated to each of the 10 indicators are obtained from literature looking at floods and landslides risk assessment procedures applied to historic buildings and cultural landscapes (Granger et al., 1999; D`Ayala et al., 2006; Paupério et al., 2012; Kappes et al., 2012; Julia and Ferreira, 2021). They are reported in Table 1 where the total weight for normalization is 6.0.
Although the lack of systematic empirical data on damages caused by natural hazards over decades or centuries, makes impossible to establish a direct correlation between risk indices and damage levels (Julia and Ferreira, 2021), these risk indices allow to identify vulnerability trends among a homogeneous group cultural heritage, and to highlight vulnerability distribution over regions.
2.2 The application of the IBA
Based on the literature survey, an IBA (Mebarki et al., 2012; Stephenson and D’Ayala, 2014; Miranda and Ferreira, 2019), which integrated the most frequently used physical and cultural indicators (or conditioning factors), was used to assess the churches’ vulnerability and risk. The socio-economic aspect of the vulnerability – linked to number of fatalities, monetary damages to urban infrastructures and/or damages on surrounding ecologic systems – was considered not relevant to assess potential value and significance losses referred to the medieval churches as instead they were the physical and cultural indicators.
The IBA indicators in the sensitivity component were assessed through visual and documentary review assessment techniques looking at the material and data collection:
Material: Stone for the foundations and Scots pine for the building structure. The material is homogeneous in all the group of the 28 SCs. The sensitivity is classified looking at the literature (Miranda and Ferreira, 2019; Ferreira and Santos, 2020) based on wood’s properties that being an absorbent material show significant decay levels when in contact with flowing and stagnant water in case of flood events; and that depending on material tensile strength, heterogeneity and anisotropy, or depending on connections between the structural elements in the construction may show inadequate structural response during landslide events. In the assessment of this indicator, the score remains the same within the group of churches, but it changes between flood and landslide risk as reported in the above quoted literature (see results in section 3.2).
Condition of the building envelope: The level of maintenance and preservation of the building envelope was investigated based on the reports produced after the SCPP.
Number of storey: A single-storey building is here considered. In case of floods the sensitivity of this indicator may be higher respect to landslides because of the type, level and height of ground floor and basements.
Age: Dates on the SCs’ age were collected and reported in Table 2.
Heritage status: The level of protection of heritage buildings in Norway is here considered being homogeneous and maximum. In fact, this is an indicator reporting the cultural dimension of the significance of the SCs that has a global impact.
Heritage content: In the case of the SCs, higher sensitivity should be assigned to heritage and collections with irreplaceable value, such as those constituted by wall distemper paints and medieval religious objects.
The IBA exposure component was assessed as follows:
Wall orientation and openings; i.e. the topography was analyzed using the Google Earth pro ©, a computer program that renders a 3D representation of Earth based on satellite Copernicus imagery for Norway. The program maps the investigated locations by superimposing satellite images, aerial photography and GIS data. It allows investigating the relationship between the SC location and its surrounding topography in the landscapes from various angles extracting numerical information to categorize the levels of indicators.
Altitude: Retrieved using Google Earth pro. It is used to understand the local relief as landslide has a higher tendency to occur at higher altitude (Youssef et al., 2015). This indicator was divided in 4 levels (A: 0–125 m; B: 126–150 m; C: 251–375 m; D: 376–500 m).
Slope: This indicator is derived from the extrapolation of the elevation profile of the minimum path or distance between the SC’s location and the closest border of a flood or landslide HA as reported below. The elevation profile is constituted by a X-Y chart where X-axis displays the distance and the Y-axis the elevation. The slope in percentage is then calculated using equation (4):
The slope indicator was divided in 4 levels i.e. A: 1.0 – 2.5%; B: 2.6 – 5.0%; C: 5.1 – 7.5%; D: >7.5%. High exposure is evaluated when the slope is negative i.e. when the SC altitude is lower than HA and the curvature (i.e. rate of change in slope gradient in a specific direction) is appropriate for triggering the hazard phenomena, while exposure is low when slope is positive. Distance between the SC and the HA. The analysis of the distance between the churches’ locations and the border of the closest HA is categorized in 4 classes as reported in the legend in Figure 3 where A: dSC-HA>300 m out of HA, B: 150 < dSC-HA <300 m out of HA, C: at the boundary of HA with dSC-HA<150 m and D: into the HA with dSC-HA<150 m. The HA is visualized with color codes within the danger maps developed by the NVE on the basis of their hazard inventory DB (https://www.nve.no/map-services/). This is a national data set that shows which areas may be exposed to hazards.
Generally, when assessing a flood or landslide hazard, the first information to collect are their probability or return period and their extent (both analyzing historical events or modeling them), usually depicted in terms of extent maps. As an example, in case of flood, the first step is to assess discharge calculated by means of frequency analysis, based on data from existing stream flow gauging stations that are then extrapolated to ungagged parts using regionalization techniques for three specific return periods, as required by the EU FD. In the case of hazard maps produced by NVE, they have a resolution of 0.6 km × 0.6 km and are developed using historical data of past floods and landslides events (i.e. accounting for the frequency and intensity of past events). In the second step, the discharge needs to be decoded into water level, usually done through hydraulic/hydrodynamic models. Historical knowledge, e.g. from water lines, is used to calibrate the models. Finally, over the last step, the geohazard affected area is determined by combining water level with a digital elevation model (DEM), thus creating a danger map as done by NVE in Norway. Specifically, a flood or landslide danger map, use matrices or formulas to link flood parameters (e.g. water depth, return period) to categories of “qualitative” measure of danger. The formula used by NVE to measure the flood danger is:
HA containing visual information on the consequences of flood and landslide in terms of extension in combination with the hazard information for both floods and landslides at the 28 sites locations (see Appendix 2). Thanks to this specific indicator (see Figure 2) it is possible to transform the vulnerability-index based approach in a risk-based approach.
As already mentioned, each indicator in Figure 2 can be ranked using a 4-level scale (i.e. from A to D) depending on the characteristic level recognized in each SC. A-level has the lowest factor i.e. 0.25, while D the highest i.e. 1.00 with B and C levels having intermediate grades of 0.50 and 0.75, respectively.
The single and the independent multi-hazard risk assessment were conducted for the 28 SCs’ locations using equations (1)–(3) (with j ranging from 1 to 28). The risk indices allowed identifying vulnerability trends among the homogeneous group of the SCs, to rank the top five SCs at single or multi-hazard risk and to the highlight vulnerability distribution over regions.
3. Results
3.1 Stave churches architectural features and geographical characteristics
As mentioned in the DRR (UNISDR, 2015) policy makers, stakeholders and heritage institutions need to raise awareness on natural hazards, to collect and document heritage buildings significance, preservation and maintenance conditions, response and adaptation needs as well as to effectively recognize and prioritize risk preparedness. Make research and data openly available is an existing barrier which need to be overcome. As example of such barrier, existing data on knowledge and published outputs related to the SCs are largely inaccessible to the wide international (English speaking) research community due to local language (mainly Norwegian) and/or format (i.e. white and grey literature) used. In fact, beside the book by Bakken et al. (2016) written upon completion of the SCPP, few peer reviewed international works generally exist on SCs (Freeman et al., 2021, 2023, Olstad et al., 2020, Berg, 2018, Espedal, 2017, Hamre et al., 2007, Egenberg et al., 2003, Olstad, 1994; Christie, 1978) and none of them is focused on the whole group of still existing 28 SCs. This is the reason why the first part of the results section aims to homogeneously collect and report all together existing information on architectural features, conservation status and geographical attributes for the whole group of SCs. This information is accompanied by, as much as possible complete, 2D sections collection from existing drawings and novel 3D drawn sketches created for this contribution.
The SCs were built between the 1st and the 14th centuries, and they are in the Norwegian regions of Vestland, Vestfold Telemark, Viken, Innlandet, Møre Romsdal and Trøndelag (Figure 4). Most of the churches are located near water sources with 13 churches located near a river, 5 churches near a lake, 3 churches near both a river and a lake, 4 churches near a fjord and 3 near the sea with the Grip SC (ID 6 in Table 2) sited on a small island. The altitude of the location where the churches are placed varies between ca. 20 a.m.s.l. till ca. 580 a.m.s.l.
The churches are grouped in categories according to their structural characteristics as highlighted in literature (Bakken et al., 2016): “simple”, “møre type”, “center post”, “elevated section” and “standalone”. The “simple” and the “møre type” churches are both characterized by a rectangular nave, but the “møre type” introduced intermediate posts. The Møre type is peculiar of the Norwegian coastal region of Møre. The center post churches have a large center post connecting the foundations and the roof, while the “elevated section” SCs have a more complex construction with raised nave churches. The “standalone” churches do not fit in any of these categories, and, from our overview, they are located at higher altitude (Figure 5b). Most of the churches belong to the “elevated section” category that is also represented by churches with ancient original elements (Figure 5a).
The churches have undergone several modifications, including refurbishment, demolition and reconstruction, displacements, expansions and reconstructions of some parts. Some churches, i.e. the Garmo (ID 4), the Gol (ID 5) and the Haltdalen (ID 7) SCs, were disassembled, moved and reconstructed in the Maihaugen open-air museum in Lillehammer, the Norwegian Museum of Cultural History at Bygdøy in Oslo and the Trøndelag Folk Museum in Trondheim, respectively. Other churches were extensively refurbished and later brought back to the presumed original status, e.g. the Heddal SC (ID 9), which was reconstructed in the 1950s to return it to its supposed medieval design.
Several churches presented problems in the foundations which were restored or replaced, such as Hegge (ID 10) in 1924 or Haltdalen (ID 7) in 2015 within the SCPP, in other cases the churches were even straightened, e.g. two times for Lomen (ID 17) in 1600s and 1800s, or even elevated of 30 cm on new foundations in the case of Undredal (ID 25). Few remained mostly original. Borgund (ID 1), Kvernes (ID 15) and Reinli (ID 19) are well preserved, the restoration work for these churches were minimal (Bakken et al., 2016). While Urnes (ID 26), the only one inscribed in the UNESCO world heritage list since 1979, is one of the oldest SCs reporting an exceptional example of craftsmanship capable to contribute to the understanding of the development of this specific building tradition. More details on the state of preservation of the churches are displayed in Table 2.
3.2 Flooding and landslide risk
Using the available data collected on the SC and following the IBA, illustrated in section 2, the risk assessment for both floods and landslides was conducted for the whole group of the 28 SCs except for Grip. For the latter SC, located on the small island of Grip, it was not possible to retrieve topographic information using Google Earth probe nor the HA from the NVE maps. Therefore, the level of completeness of the provided information is greater than 96%.
The matrices of risk components for floods and landslides events are reported in Figure 6 left and right respectively. In the matrices, lines (i-index in equations (1) to (3)) report the indicators in Figure 2; columns (j-index in equations (1) to (3)) report the 28 SCs ID codes. The colored (i; j) elements are constituted by weighted indicators (i.e. indicators multiplied by their weights reported per each church). The color code shows the main risk factors (i.e. dark red color) or the single resilient factors (i.e. the unfavorable slope that cannot make possible downslope movement of water, soil and rocks toward the SC and highlighted in dark blue color) which may prevent the triggering of the hazard event. Concerning the flood risk ranking, main causal factors are the HA and the wall orientation linked to the exposure and hazard, while age is the most sensitive factor. Interestingly the factor that prevents the hazards is the slope as the majority of the SCs were built at the higher altitude than water bodies. Concerning the landslides risk ranking, principal causal factors are HA at high altitude and appropriate slopes and curvature to trigger the phenomena, while main sensitive factors are the material mechanical properties. Circa 40% of the SCs (white and blue shadows colors) were built on the top or far from embankments formed by earthy material moved by past landslides.
The overview of the single-hazard risk rank (in percentage from 0% to 100%) after the calculation done with equations (1) and (2) is shown in the plots in Figure 7a and b. The low (L), medium (M) and high (H) levels of risks are arbitrary with L < 33%, 33% < M< 66% and H>66%. The independent multiple-hazard risk rank as calculated by equation (3) (in percentage from 0% to 200%) are set at L<66%; 67%<M<132%; H>133% and reported in Figure 7c following a similar procedure as that used in the selection of risk level thresholds in the climate for culture maps (Leissner et al., 2015). This procedure, although being arbitrary, is however meaningful as it supports decision makers in taking – not an absolute – but a relative-based decision. This means that the decision of planning and implementing mitigation measures for DRR is based on the different level of risk between the stave church under examination and the average risk of all the other SCs at national scale, regional scale or within the same climatic area (relative-based decision over space). Similarly, a relative-based decision over time support decisions based on any detected change in floods or landslides risk over consecutive temporal uses of the IBA procedure for the same stave church.
Based on these levels, the top-five ranked churches at single and independent multi-hazard risk were reported in Figures 8 and 9 respectively.
Flooding: In Figure 7a, 19% of the churches (5 over 27) are at low risk, 78% (21 out of 27) at medium and only one (ID 3, Flesberg) at high risk. The two top lines in Figure 8 report the details of the HA as extrapolated by the NVE danger maps for the top five ranked churches prone to flood hazards. Flesberg is the church at most risk as it is located into the HA with an orientation and a slope respect to the surrounding landscape that helps the water runoff in case of river overflood. The other four churches are at the limit between M/H risk level. Heddal (ID 9) is located in a flood danger area which experienced past floods and being flat may retain overflooded water for longer. Høre (ID 13), similarly to Flesberg, is on the HA boundaries, the area has a proper slope and curvature for water runoff. Finally, both Røldal (ID 23) and Øye (ID 28), despite being located inside HA, have a lower level of risk as the surrounding landscape do not incentive the water runoff.
In Norway, looking at expected climate and natural hazards change scenarios, the NVE (Norwegian Ministry of Climate and Environment, 2013) indicates that pattern of change for floods is subject to a decrease in term of annual mean flood within northern and inland areas (e.g. inland areas in the Innlandet region and in Vestfold-Telemark see Figure 4f), where the churches of Høre and Heddal are located; and to an increase in western and south-western regions and coastal regions (e.g. in Trøndelag, Møre-Romsdal and Vestland) (Lawrence and Hisdal, 2011; Wilson et al., 2011). Therefore, especially in the area surrounding Røldal, an increase in awareness about such risk is strongly needed.
In addition, the Norwegian Ministry of Climate and Environment (2013) and the Norwegian Centre for Climate Services (NCCS) (Hanssen-Bauer et al., 2018) warn on the possible severe increase of flooding events in areas where rain floods already occurred. In fact, in river systems that are dominated by rain floods, the magnitude of future floods is projected to increase by up to almost 60% (under RCP8.5 scenarios). More frequent and stronger rainfall events may challenge small, steep rivers and urban areas (Norwegian Ministry of Climate and Environment, 2013; Hanssen-Bauer et al., 2018). Therefore, monitoring changes in topographic indicators as considered in this IBA (altitude, slope and curvature, HA surrounding the body waters catchment) will become increasingly significant.
Finally, both precipitations and snowmelt play a key role in understanding water flows; higher temperatures may lead to a reduction of snow storage with consequent precipitation felt as rain instead of snow and earlier spring floods. Even the frequency of late autumn and winter floods might increase (Lawrence and Hisdal, 2011; Wilson et al., 2011; Norwegian Ministry of Climate and Environment, 2013; Hanssen-Bauer et al., 2018).
Landslides: This contribution is focused on the analysis of danger of Earth slides – including flood slides and smaller rockslides often triggered by intense rainfall. In Figure 7b, 19% of the churches (5 over 27) are at low risk, 56% (15 out of 27) at medium and 26% (7 out of 27) at high risk. The two bottom lines in Figure 8 show the HA extension for the top five ranked churches prone to landslide hazards. Uvdal (ID 27) is the church at most risk (88%) as it is located at the bottom of a HA with steep topography, where rapid rainfall may initiate mass wasting. Torpo (ID 24) and Ringebu (ID 20) have a slightly lower risk level than Uvdal being at the border of the HA i.e. the expected area where rocks and soil materials accumulate in case of downslope movements. Finally, Øye (ID 28) and Borgund (ID 1) are in high-risk class due to their proximity with the HA, although the steepness of the surrounding slopes is less pronounced. This helps in maintaining the stability between the shear force and the normal ground force. It is when the slope angle becomes steeper, or the Earth material is weakened, for example by a variation in water content caused by precipitations that the shear force exceeds the normal force and downslope movement occurs. Therefore, the soil water content, located in pore spaces in sediments or rocks between the grains, is a factor that needs to be monitored in proximity of the SCs at high risk. Another factor which may influence shear strength is planes of weakness in sedimentary rocks (e.g. existing bedding planes parallel to the slope).
In the far future (2071–2100) respect to the recent past (1991–2020), as already explained above, the increase in frequency and amount of precipitation might trigger the risk of landslides, especially in steep terrain, even in areas with no impacts occurred previously (Norwegian Ministry of Climate and Environment, 2013; Hanssen-Bauer et al., 2018). Higher anomalies in precipitation are expected in the months of February and March for the SCs at high risk highlighted in Figure 8 Ringebu, Torpo and Øye. This will trigger a larger soil moisture anomaly during mid-April and mid-May. Earth slides are only one of the slides sensitive to climate change which may impact the Norwegian regions. In facts, the expected increase of temperature may lead to shorter snow seasons which may affect the frequency of avalanches.
Dry snow avalanches may decrease due to the altitude of the snow limit, while wet snow avalanches and slush slides may increase, with events occurring in areas not previously affected (NGI, 2013; Jaedicke et al., 2008). Permafrost thawing may also trigger large rockslides in the near future, however there is paucity of scientific evidence to conclude that climate change will have an effect on it.
Independent multi-hazard risk: Figure 7c reports the independent multi-hazard risk as the sum of the outcomes of the single-hazard risk results (equation 3) obtained using the IBA scheme. However, multi-hazard risk in real world observations is not just the sum of its components but it has in addition interacting terms. Nevertheless, as stated by Tyagunov et al. (2005) and by Kappes et al. (2010), nowadays it is still difficult to understand and model potential interaction entirely. This is due to several aspects. From one side single-hazard risk assessment approaches (e.g. as the IBA) are well known and correctly identify and integrate major processes and parameters concerning the general setting which favors hazards. From the other side, communities working on multiple-hazard risk still have a lack of (1) standard definition, terms and units, and (2) procedure to directly compare the multi-hazards interactions in terms of intensity, return period and parameters of influence on elements at risks (e.g. extent, predictability, time of onset and duration). Nevertheless, it is still possible to underline some aspects of interconnection between floods and landslides risk at the SC locations: (1) in areas with hazard overlapping, it is expected that one hazard may modify the general setting of another process thus requiring a repetition of the risk assessment procedure. Identified influences between the single-hazard risk are debris flows that may change riverbed morphology; rock falls that – depending on increased slope roughness and supply of material – may increase load and material accumulation in riverbed; landslide that – depending on alteration of surface roughness and supply of material – may change river course, and finally floods that may be caused by remobilization of material and erosion or saturation of landslide deposits. (2) Beside the interconnection or influence, amplification as result of the cascading hazard effect or due to the coincidence (i.e. hazard that occurs at the same place simultaneously) is an important aspect to consider. As an example, reported to the SC, amplification may be triggered by the same nonhazard event (e.g. heavy rainfall) that in turn may cause two hazards, i.e. floods and debris flows. A coherent analysis scheme (e.g. IBA) is a fundamental precondition for making this consideration in terms of multi-hazard relationships.
Figure 9 reports the five SCs at higher independent multi-hazard risk (i.e. both flood and landslide risk). The SC at high multi-hazard risk is Øye (ID 28) as it is in the HA for the two hazards, while Torpo (ID 24) has a high-medium multi-hazard risk level mainly caused by its location into the HA for earth landslides.
4. Discussion
The EU FD over these last 15 years has played a leading role in developing a common conceptual framework to create flood mapping and flood risk management (De Moel et al., 2009). However, limitations remain in comparing risk maps respect to hazard maps. This is because risk maps are based on the use of many indicators implemented mainly to answer specific management questions arose by consequence of catastrophic events; in comparison hazard maps are based on few indicators that are better standardized (e.g. flood extent or water depth). The setting up of common management plans remain therefore difficult to be achieved, especially when comparing plans between EU and nonEU countries. In this paper NVE’s maps were used, which consider the potential maximum risk (i.e. worst case scenario) leading to maximum negative consequences. This maximum degree of caution allows decision makers and heritage managers to acquire risk awareness to face the worst case scenario and plan and implement appropriate measures for its DRR.
This contribution recognizes the importance of risk reduction through climate action that means that DRR can be successful only through a coherent understanding of the hazard linked with the climate change impact on which basis to fund possible coherent adaptation solutions. This procedure is in line with the UNDRR that together with other UN system agencies, are making a global push to ensure that existing national DRR strategies (e.g. strategies funded on the existing NVE danger maps) are coherent at country level with climate change policies globally and regionally. This focus on coherence is nowadays a vital step in ensuring to do not waste economic, social and environmental resources and above all in assuring the best possible preservation to the 28 medieval SCs.
The contribution tries to improve the IBA framework filling the paucity highlighted in the literature review (section 1.2) that is the lack of vulnerability indicators created specifically for immovable cultural heritage, and for movable heritage objects preserved inside, considering both their tangible and intangible value. One of the recognized IBA limitations is the difficulty of calibrating its sensitivity component as for example the condition of the building envelope. This could be done theoretically conducting precise in situ testing and monitoring campaigns on the envelope using instrumentation that does not affect the integrity of the heritage material. Notwithstanding this type of quantitative assessment may not be the most suitable one when coupled with IBA. It can work better in assessing semi-quantitatively, the consequences of floods and landslide which can lead to loss of historic buildings, including their interiors and collections, as well as to the modification of cultural landscape, leading to disappearance or alteration of their intangible significance which contributes to provide the collective memory to the local community. The IBA proposed in this paper has introduced the new indicator “Heritage Content” which allows prioritizing both DRR and adaptation planning actions in order to preserve collections of irreplaceable value. To promote DRR and improve the adaptive capacity of heritage sites it is suggested the integration of the proposed IBA with the validation of protective measures from flood and landslide events. Knowing the outcomes of the IBA framework, site managers and stakeholders involved in heritage preservation might want to investigate the efficiency of defenses that are already in place or even start to plan for new adaptation options, for example: dredging, watercourses regularization and requalification, retaining walls, sustainable drainage systems as water retention, green roof, permeable pavements, ponds and dry basins, reforestation to name a few, especially in light of potential exacerbated effects of climate change.
There is a paucity of literature which considers the effect of climate change on future flood and landslide hazards although the expected increasing risk that must be taken into account, especially in the second six-year cycle of the EU FD. This lack of knowledge is expected to increase even more the pressure on governments in terms of investment to structural and nonstructural protection measures for safeguarding people, cultural heritage, natural, built and industrial environment. In Norway, the NVE has developed its second strategy for climate change adaptation (NVE’s strategy for climate change adaptation 2015–2019 – summary in English) that covers NVE’s areas of responsibility. This includes how to use instruments such as flood and landslide hazard mapping, land use planning and protection measures as tools in climate change adaptation. The general awareness regarding climate challenges has increased. The influence of climate change on floods (Lawrence, 2016) is now included in flood hazard maps. Relevant knowledge has been incorporated in guidelines. For example, how to take climate change into consideration in design flood estimates is included in the Dam Safety Guidelines. Particularly sensitive dams have been identified, and protection against flood and landslide hazards is included in the guideline “Floods and landslides in land use plans” (NVE, 2014). In a newly developed cost/benefit tool to assess and prioritize between protective flood and landslide measures, climate change effects are included. The observed and projected climate development in particular calls for measures to protect against floods, erosion and landslides in small, steep, mass-transporting rivers with a large potential for damage. A particular guideline for floods in small rivers has been issued (NVE, 2016a).
The availability of flood hazard maps (extend and danger maps) online, as in the case of the NVE database for Norway, is the proof that the EU FD objectives is raising public awareness toward geohazards damages potential and toward the need of developing tools for enhancing coping capacity to locally handle hazardous events. Further research could improve knowledge on early warning and on the preparedness for cultural heritage, which remain still limited:
Climate change challenges cultural heritage sites in many ways; regulations and planning system, local policies for climate mitigation and climate adaptation need to be improved to strengthen the role of cultural heritage values in urban development. For instance, the role of (urban) nature in absorbing surplus water from heavy precipitation is an important service that contributes to protecting cultural heritage sites and cultural heritage environments.
For the most vulnerable churches, as the SCs, hazard maps should be developed based on local conditions. Advice on appropriate adaptation measures should be provided with reference to local examples.
Norway is a very mountainous country with rather small river basins and it is sparsely populated. Making flood inundation maps covering all the flood prone areas regardless of population density will be both time and money consuming without giving much benefit. To identify a useful threshold of significant potential flood risk is therefore of interest. As for the environment, cultural heritage is not a factor that points out sites of significant flood risk during the preliminary flood risk mapping phase. Cultural heritage sites that are of significant value should be identified in more detailed flood hazard and flood risk maps.
In the changing climate, the type of landslide most likely to increase in frequency is “wet” landslide, such as debris flow and slush avalanches. These types of landslides are usually not considered when planning new roads. “Wet” avalanches are often triggered by human activity in the release area. Prevention methods are drainage, open or closed control dams and/or bridges in avalanche course, deflectors, sedimentation basins, alternative drainage paths in the runout area and moving the road. Alternative drainage paths are an important remedial measure for sediment transport along streams and rivers, for ensuring operative drainage systems. A risk assessment of existing roads with respect to increased erosion and sediment transport should be carried out, especially when these are touristic routes or are connecting valuable cultural heritage sites.
5. Conclusions
The still existing 28 SCs constitute a homogeneous group of heritage buildings globally recognized to be outstanding examples of traditional Scandinavian wooden architecture embedding traces of Celtic art, Viking traditions, Middle Age Western European culture and – in their spatial structures – traces of Romanesque. These ancient wooden buildings are also outstanding as they are example of large-scale reuse of both decorative and constructive elements originating from earlier SCs construction and assets in a single building. For this high significance they need special maintenance, preventive conservation efforts, including continuous monitoring, survey and research programs. Over the last decades, the Norwegian directorate of cultural heritage with the 15 years long SCPP (2000–2015) and the Norwegian Research Council with the funds` opportunity provided to the International Research project Symbol – Sustainable Management of Heritage Buildings in a long-term perspective (2018–2022) have worked in this direction.
However, these efforts of studying and taking care of the SCs are still limited. One of the weaknesses that this study has underlined is the paucity of homogeneous and complete data and survey about the 28 churches architectural features (e.g. sections and plans from in situ survey) to the authors’ knowledge recent surveys are not available as open access information, in addition most of the identified literature is white, grey or written in Norwegian language. The plan scheme used as reference in this research were mostly historical (1800–1900s) and might be not up to date with the current churches’ conditions. The digital recording of cultural heritage resources, especially those in danger from natural hazard and climate change threats, is one of the adaptation solutions promoted by ICOMOS and UNESCO. Laser scanner technologies or other techniques useful to digitally record cultural heritage should be used to preserve its memory for future generations in case of loss and/or for reconstruction after a disaster event. This is the case of the ongoing project supported by the Norwegian Directorate of Cultural Heritage (Riksantikvaren) and the National Trust of Norway (Fortidsminneforeningen) that is surveying and digitally reconstructing a total of 28 preserved Norwegian SCs.
The main objective of this contribution is the assessment of the danger from natural hazards on the whole group of 28 SCs. Results have shown that most of the churches are assessed to be at medium risk of flooding (e.g. 78%, 21 out of 27), while in case of landslides the risk increases to high level for the 26% of the churches (7 out of 27) and remains at medium level for the 56% (15 out of 27). There is evidence that the SCs may be endangered by flooding and landslide. Furthermore, these impacts might speed up under climate change; more research is needed to understand how they might impact cultural heritage resources in a changing climate. This paper highlights the key indicators which are crucial to monitor over time in order to prevent hazards at the SCs locations. The details of the ranked top five churches into or in proximity of HA put the basis for a bigger question which needs to be supported and answered by further research i.e. how to plan, install and continuously monitor changes in landscape conditions (e.g. slope steepness, soil grains and water content) close to these churches.
In this direction, the paper contributes to a better understanding of place-based vulnerability with local mapping dimension also considering future threats posed by climate change. The results highlight the danger at which the SCs of Røldal, in case of floods, and of Ringebu, Torpo and Øye, in case of landslide, may face and stress the urgency of increasing awareness and preparedness on these potential hazards.
These first valuable disaster risk information, besides showing the needs for additional research, easily convey floods and landslides awareness. The use of these maps and information can be incorporated into the SCs preservation frameworks and DRR management practices. Fostering communication, engagement and feedback within stakeholders and communities to create a bottom-up participatory process is highly suggested.
Figures
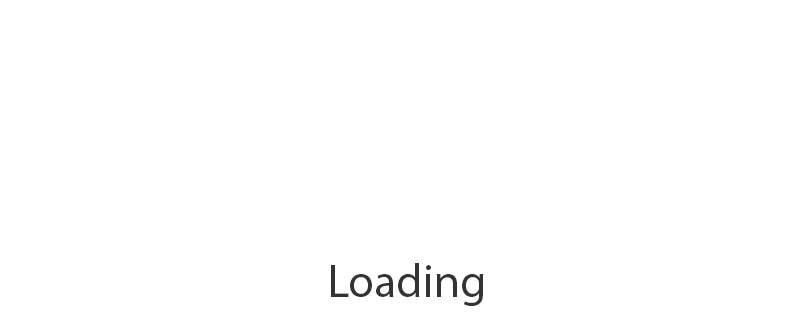
Figure 2
IBA scheme for floods and landslides risk built on Miranda and Ferreira (2019) and Ferreira and Santos (2020)
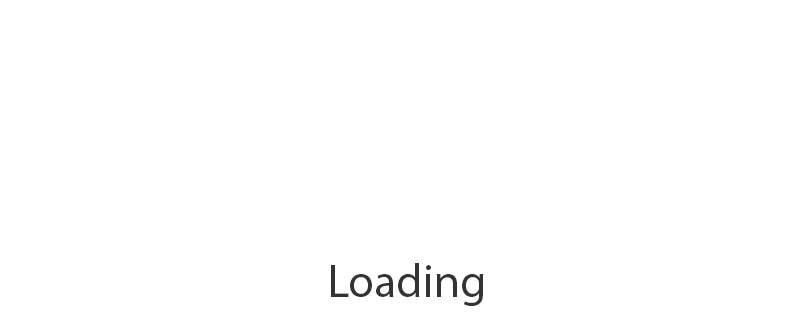
Figure 5
(a) SC architectural type compared to the year of construction. (b) SC architectural type analyzed by altitude (meter a.m.s.l.). (c) Year of construction and subsequent refurbishment according to the IDs of the SCs as reported in Table 2. (d) SCs' type according to IDs and grouped as per Norwegian regions
Weight Wflood,i and Wlandslide,i for each of the ten indicators (Ii) that can be used for assessing flood and landslide risks as reported in Figure 2
Material | Condition | Storey | Age | Status | Content | Altitude | Slope | dSC-HA | Orientation | |
---|---|---|---|---|---|---|---|---|---|---|
I1 | I2 | I3 | I4 | I5 | I6 | I7 | I8 | I9 | I10 | |
Wflood,i | 0.6 | 0.4 | 0.8 | 0.6 | 0.2 | 0.4 | 0.2 | 1.0 | 1.0 | 0.8 |
Wlandslide,i | 1.0 | 1.0 | 0.2 | 0.2 | 0.2 | 0.4 | 0.6 | 1.0 | 1.0 | 0.4 |
ID, name, latitude and longitude, region, altitude, site description, category, preservation state, plan and 3D draw per each of the 28 SCs
![]() |
![]() |
![]() |
![]() |
![]() |
![]() |
Plan scheme references
N | Stave church name | Plan scheme based on the following references |
---|---|---|
1 | Borgund | kulturminnebilder.ra.no – Illustration by: Franz Wilhelm Schiertz KID 83933 (1837) |
kulturminnebilder.ra.no – Illustration by: Georg Andreas Bull KID 83933-1 (1850–1870) | ||
kulturminnebilder.ra.no – Illustration by: Herman Major Schrimer, Riksantikvaren KID 83933-1 (1861) | ||
Andersen (2019) – Plan. Etter Wilhelm Lübke, Max Semrau, Grundriss der Kunstgeschichte, 1908 | ||
Bakken et al. (2016) – Based on a drawing by Håkon Christie | ||
Bakken et al. (2016) – Lithograph published in Dahl’s book from drawings by J.C.Dahl’s pupil Franz Wilhelm Schiertz | ||
2 | Eidsborg | kulturminnebilder.ra.no – Illustration by: Johan Meyer KID 84071-1 (1890–1900) |
5 | Gol | kulturminnebilder.ra.no – Illustration by: Georg Andreas Bull KID 84275-1 (1800) |
Bakken et al. (2016) – Drawing: Jan Michael Stornes | ||
7 | Haltdalen | Clare Casassas (2019) |
8 | Hedalen | kulturminnebilder.ra.no – Illustration by: Carl Berner KID 84512-1 (1902) |
kulturminnebilder.ra.no – Illustration by: Georg Andreas Bull KID 84512-1 (1853) | ||
kulturminnebilder.ra.no – Illustration by: Johan Meyer KID 84512-1 (1893) | ||
9 | Heddal | kulturminnebilder.ra.no – Illustration by: Grontoft, Hjørdis KID 84513-1 (1896) |
Bakken et al. (2016) – Illustration from J.C. Dahl’s book on Norwegian stave churches (1830s) | ||
10 | Hegge | kulturminnebilder.ra.no – Illustration by: Henrik Bull, Riksantikvaren KID 84516 (1912) |
11 | Hopperstad | kulturminnebilder.ra.no – Illustration by: Georg Andreas Bull KID 84627-1 (1954) |
kulturminnebilder.ra.no – Illustration by: Peter Andreas Blix KID 84627-1 (1870–1890) | ||
Bakken et al. (2016) – Drawing: Jan Michael Stornes | ||
Lehne et al. (2019) | ||
12 | Høyjord | Bakken et al. (2016) – Drawing: Jan Michael Stornes |
14 | Kaupanger | kulturminnebilder.ra.no – Illustration by: Georg Andreas Bull KID 84766 (1854) |
Bakken et al. (2016) – Drawing: Jan Michael Stornes | ||
15 | Kvernes | Olstad et al. (2020) – Source: Illustrated guide to the stave Church by the architect Heinrich Joachim Sebastian Karsten (1873–1947) in 1900 |
16 | Lom | kulturminnebilder.ra.no – Illustration by: Christian Christie KID 84322-1 (1860) |
17 | Lomen | kulturminnebilder.ra.no – Illustration by: Georg Andreas Bull KID 84323 (1855) |
kulturminnebilder.ra.no – Illustration by: Utne, R.KID 84323-1 (1909) | ||
18 | Nore | kulturminnebilder.ra.no – Illustration by: Einar Oscar Schou, Riksantikvaren KID 85174 (1901) |
Bakken et al. (2016) – Drawing by Einar Oscar Schou, 1901 | ||
Bakken et al. (2016) – Drawing: Jan Michael Stornes | ||
19 | Reinli | kulturminnebilder.ra.no – Illustration by: Georg Andreas Bull KID 85281-1 (1853) |
20 | Ringebu | kulturminnebilder.ra.no – Illustration by: Hakon Thorsen KID 85295 (1884) |
21 | Rollag | Bakken et al. (2016) – Drawing: Jan Michael Stornes |
24 | Torpo | kulturminnebilder.ra.no – Illustration by: Georg Andreas Bull KID 85649 (1855) |
kulturminnebilder.ra.no – Illustration by: Unknown KID 85649-1 (N.A.) | ||
26 | Urnes | kulturminnebilder.ra.no – Illustration by: Einar Oscar Schou KID 85729-1 (N.A.) |
kulturminnebilder.ra.no – Illustration by: Unknown KID 85729-1 (N.A.) | ||
kulturminnebilder.ra.no – Illustration by: Georg Andreas Bull KID 85729-1 (1854) | ||
Bakken et al. (2016) - Drawing: Jan Michael Stornes | ||
27 | Uvdal | kulturminnebilder.ra.no – Illustration by: Thorvald Borgersen, Riksantikvaren KID 85738 (1901) |
Bakken et al. (2016) – Based on a drawing by Håkon Christie | ||
Bakken et al. (2016) - Drawing: Jan Michael Stornes | ||
28 | Øye | kulturminnebilder.ra.no – Illustration by: Unknown KID 85935-2 |
Flood and landslide risk for the 28 stave churches
![]() |
![]() |
![]() |
![]() |
![]() |
![]() |
![]() |
Notes
Urnes SC, https://whc.unesco.org/en/list/58
Reports on the SCPP, in Norwegian, available at: https://ra.brage.unit.no/ra-xmlui/handle/11250/176302?locale-attribute=en
Conflicts of interest: The authors have no conflict of interest to declare.
References
Andersen, H.A. (2019), De norske stavkirkenes svalganger i et internasjonalt perspektiv”, Konsthistorisk tidskrift/Journal of Art History, Vol. 88 No. 2, pp. 95-112, doi: 10.1080/00233609.2019.1634640.
Anker, L. (2016), “What is a stave church?”, in Bakken, K. (Ed.), Preserving the Stave Churches. Craftmanship and Research, Pax Forlag, Riksantikvaren.
Bakken, K., Greenhow, I. and Ostling, G. (2016), Preserving the Stave Churches: Craftsmanship and Research, The Norwegian Directorate for Cultural Heritage, Print Best OU, Estonia.
Berg, F. (2018), “Wear and tear of world heritage: preventive conservation and tourism in Norway’s stave churches”, Studies in Conservation, Vol. 63 sup1, pp. 320-322.
Brimblecombe, P., Grossi, C.M. and Harris, I. (2011), “Climate change critical to cultural heritage”, in Gökc, H., Ekus, U. and TürkerLamoreaux, J.W. (Eds), Survival and Sustainability, Springer Berlin Heidelberg.
Cambiaggi, L. and Berardi, R. (2019), “Investigation on the damages induced by slope movements on historic buildings: the case of San Nicolò di Capodimonte church in Liguria”, Proceedings of the XVII ECSMGE-2019, Geotechnical Engineering Foundation of the Future, doi: 10.32075/17ECSMGE-2019-0180.
Christie, H. (1978), “Lom stave church”, World Archaeology, Vol. 10 No. 2, pp. 192-203.
Cigna, F., Liguori, V., Del Ventisette, C. and Casagli, N. (2013), “Landslide impacts on Agrigento’s cathedral imaged with radar interferometry”, in Margottini, C., Canuti, P. and Sassa, K. (Eds), Landslide Science and Practice, Vol. 6, pp. 475-481, doi: 10.1007/978-3-642-31319-6_63.
Clare Casassas, C.R. (2019), “The Norwegian Stavkirke”, Master Degree thesis in Art History, Universitat de Girona, p. 47, available at: http://hdl.handle.net/10256/16815
Colette, A. (2007), “Climate change and world heritage”, Report on predicting and man-aging the impacts of climate change on World Heritage and Strategy to assist States Parties to implement appropriate management responses, UNESCO World Heritage Centre, Paris.
Cuca, B. (2020), “Copernicus Sentinel imagery for more risk-resilient historic cities in coastal zones: contribution to the monitoring of Albenga archaeological site and delta of river Centa”, Applied Geomatics, Vol. 14 No. 1, pp. 67-78, doi: 10.1007/s12518-020-00340-5.
De Moel, H., van Alphen, J. and Aerts, J.C.J.H. (2009), “Flood maps in Europe – methods, availability and use”, Natural Hazards and Earth System Sciences, Vol. 9, pp. 289-301, doi: 10.5194/nhess-9-289-2009.
D’Ayala, D., Copping, A. and Wang, H. (2006), “A conceptual model for multi-hazard assessment of the vulnerability of historic buildings”, in Lourenco, P.B., Roca, P., Modena, C. and Agrawal, S. (Eds), Structural analysis of historical constructions; possibilities of numerical and experimental techniques. Proceedings of the 5th int. conf., New Delhi, pp. 121-140.
EEA (2017a), “Climate change, impacts and vulnerability in Europe 2016. An indicator-based report (EEA report no 1/2017)”, European Environment Agency, Copenhagen.
EEA (2017b), Climate change adaptation and disaster risk reduction in Europe – Enhancing coherence, of the knowledge base, policies and practices (EEA report no 15/2017), European Environment Agency, Copenhagen.
Egenberg, I.M., Holtekjølen, A.K. and Lundanes, E. (2003), “Characterisation of naturally and artificially weathered pine tar coatings by visual assessment and gas chromatography-mass spectrometry”, Journal of Cultural Heritage, Vol. 4 No. 3, pp. 221-241.
Espedal, K.J. (2017), “From stone to Norwegian wood”, International Journal of Computational Methods and Experimental Measurements, Vol. 5 No. 6, pp. 985-996.
Ferreira, T.M. and Santos, P.P. (2020), “An integrated approach for assessing flood risk in historic city centres”, Water, Vol. 12, p. 1648.
Figueiredo, R., Romao, X. and Pauperio, E. (2020), “Flood risk assessment of cultural heritage at large spatial scales: framework and application to mainland Portugal”, Journal of Cultural Heritage, Vol. 43, pp. 163-174.
Figueiredo, R., Romao, X. and Pauperio, E. (2021), “Component-based flood vulnerability modelling for cultural heritage buildings”, International Journal of Disaster Risk Reduction, Vol. 61, 102323.
Freeman, A.A., de Ferri, L., Mazurek, J., Andriulo, F. and Bertolin, C. (2021), “A multi-analytical approach for the characterization of seventeenth century decorative wall paintings in two Norwegian stave churches: a case study at Eidsborg and Heddal, Norway”, Applied Sciences, Vol. 11 No. 8, p. 3477.
Freeman, A.A., Fujisawa, N., Bridarolli, A., Bertolin, C. and Łukomski, M. (2023), “Microscale physical and mechanical analyses of distemper paint: a case study of Eidsborg Stave Church, Norway”, Studies in Conservation, Vol. 68 No. 1, pp. 54-67, doi: 10.1080/00393630.2021.1925487.
Granger, K., Jones, T., Leiba, M. and Scott, G. (1999), Community Risk in Cairns: A Multi-Hazards Risk Assessment, Tech. rep., Australian Geological Survey Organization (AGSO), Canberra.
Grøntoft, T. (2019), “Observed recent change in climate and potential for decay of Norwegian Wood structures”, Climate, Vol. 7 No. 2, p. 33.
Hamre, L.N., Domaas, S.T., Austad, I. and Rydgren, K. (2007), “Land-cover and structural changes in a western Norwegian cultural landscape since 1865, based on an old cadastral map and a field survey”, Landscape Ecology, Vol. 22 No. 10, pp. 1563-1574.
Hanssen-Bauer, I., Førland, E.J., Haddeland, I., Hisdal, H., Mayer, S., Nesje, A., Nilsen, J.E.Ø., Sandven, S., Sandø, A.B., Sorteberg, A. and Ådlandsvik, B. (2018), Climate in N–Rwa– 2100 - a Knowledge Base for Climate Adaptation, Norwegian Climate Service Centre (NCCS), Oslo.
Haugen, A. and Mattsson, J. (2011), “Preparations for cli’ate’change’s influences on cultural heritage”, International Journal of Climate Change Strategies and Management, Vol. 3 No. 4, pp. 386-401, doi: 10.1108/17568691111175678.
ICOMOS Climate Change and Heritage Working Group (2019), “The future of our pasts: engaging cultural heritage in climate action. Outline of Climate Change and Cultural Heritage”, available at: https://indd.adobe.com/view/a9a551e3-3b23-4127-99fd-a7a80d91a29e (accessed 6 March 2022).
IDNDR (International Decade for Natural Disaster Reduction) (1994), “Yokohama strategy and plan of action for a safer world: guidelines for natural disaster prevention, preparedness and mitigation”, available at: http://www.unisdr.org/files/8241_doc6841conte nido1.pdf (accessed 6 March 2022).
Ilieș, D.C., Caciora, T., Herman, G.V., Ilieș, A., Ropa, M. and Baias, Ş. (2020), “Geohazards affecting cultural heritage monuments. A complex case study from Romania”, Geojourrnal of Tourism And Geosites, Vol. 31 No. 3, pp. 1103-1112, doi: 10.30892/Gtg.31323—546.
Indirli, M., Razafindrakoto, H., Romanelli, F., Puglisi, C., Lanzoni, L., Milani, E., Munari, M. and Apablaza, S. (2011), “Hazard evaluation in Valparaıso: the MAR VASTO project”, Pure and Applied Geophysics (PAGEOPH), Vol. 168, pp. 543-582.
Jaedicke, C.A., Solheim, L.H., Blikra, K., Stalsberg, A., Sorteberg, A., Aaheim, K., Kronholm, D., Vikhamar-Schuler, K., Isaksen, K., Sletten, K., Kristensen, I., Barstad, C., Melchiorre, Ø.A., Høydal and Mestl, H. (2008), “`Spatial and temporal variations of Norwegian geohazards in a changing climate`, the GeoExtreme Project”, Natural Hazards and Earth System Sciences, Vol. 8, pp. 893-904.
Julia, P.B. and Ferreira, T.M. (2021), “From single- to multi-hazard vulnerability and risk in Historic Urban Areas: a literature review”, Natural Hazards, Vol. 108, pp. 93-128.
Kapović Solomun, M., Ferreira, C.S.S., Zupanc, V., Ristić, R., Drobnjak, A. and Kalantari, Z. (2022), “Flood legislation and land policy framework of EU and non-EU countries in Southern Europe”, WIREs Water, Vol. 9, e1566, doi: 10.1002/wat2.1566.
Kappes, M.S., Keiler, M. and Glade, T. (2010), “From single- to multi-hazard risk analyses: a concept addressing emerging challenges”, in Malet, J.-P., Glade, T. and Casagli, N. (Eds), Mountains Risks: Bringing Science to Society (International conference), CERG Editions, Strassbourg, pp. 351-356.
Kappes, M.S., Papathoma-Köhle, M. and Keiler, M. (2012), “Assessing physical vulnerability for multi-hazards using an indicator-based methodology”, Applied Geography, Vol. 32, pp. 577-590.
Kleinen, T. and Petschel-Held, G. (2007), “Integrated assessment of changes in flooding probabilities due to climate change”, Climate Change, Vol. 81, pp. 283-312.
Lawrence, D. (2016), “Klimaendring og framtidige flommer i Norge (Climate change and future floods in Norway)”, NVE Rapport 81/2016 (in Norwegian) (accessed 18 February 2020).
Lawrence, D. and Hisdal, H. (2011), “Report no. 5 – 2011. hydrological projections for floods in Norway under a future climate”, Norwegian Water Resources and Energy Directorate, Oslo.
Lehne, M., Mantellato, S., Aguilar Sanchez, A.M. and Caruso, F. (2019), “Conservation issues and chemical study of the causes of alteration of a part of the Stave Church in Hopperstad (Norway)”, Heritage Science, Vol. 7, p. 80, doi: 10.1186/s40494-019-0322-6.
Leissner, J., Kilian, R., Kotova, L., Jacob, D., Mikolajewicz, U., Broström, T., Ashley-Smith, J., Schellen, H.L., Martens, M., van Schijndel, J., Antretter, F., Winkler, M., Bertolin, C., Camuffo, D., Simeunovic, G. and Vyhlídal, T. (2015), “Climate for culture: assessing the impact of climate change on the future indoor climate in historic buildings using simulations”, Heritage Science, Vol. 3 No. 1, pp. 1-15, doi: 10.1186/s40494-015-0067-9.
Loreto, R.A.G., Fernandez, G.C., Jr., Liwanag, L.A.L. and Demeterio, F.P.A. (2021), “Disaster risks of the seven Filipino-Spanish churches of the Diocese of Maasin, Philippines”, SPAFA Journal, Vol. 5, pp. 1-29.
Mebarki, A., Valencia, N., Salagnac, J.L. and Barroca, B. (2012), “‘Flood hazards and masonry constructions: a probabilistic framework for damage, risk and resilience at urban scale”, Natural Hazards and Earth System Sciences, Vol. 12, pp. 1789-1809.
MICHE Project (n.d.), website, available at: https://www.micheproject.unifi.it/vp-388-our-aims.html (accessed 30 August.2022).
Miranda, F.N. and Ferreira, T.M. (2019), “A simplified approach for flood vulnerability assessment of historic sites”, Natural Hazards, Vol. 96, pp. 713-730.
Monteiro, L.R., dos Santos, C.I., Kobiyama, M., Corseuil, C.W. and Chaffe, P.L.B. (2021), “Effects of return periods on flood hazard mapping: an analysis of the UFSC Campus Basin, Florianopolis city, Brazil”, Brazilian Journal of Water Resources, Vol. 26, e9.
Moreno, M., Ortiz, R., Cagigas-Muñiz, D., Becerra, J., Martin, J.M., Prieto, A.J., Garrido-Vizuete, M.A., Macías-Bernal, J.M., Chávez, M.J. and Ortiz, P. (2022), “ART-RISK 3.0 a fuzzy—based platform that combine GIS and expert assessments for conservation strategies in cultural heritage”, Journal of Cultural Heritage, Vol. 55, pp. 263-276, doi: 10.1016/j.culher.2022.03.012.
NGI (2013), Using the Q-System—Rock Mass Classification and Support Design, NGI Publication, Oslo, p. 54.
Norwegian Map Authority (Kartverket) (2009), “Produktspesifikasjon FKB-laser Versjon 2.0 2009-02-01, Produktspesifikasjon Nasjonal modell for høydedata fra laserskanning (FKBLaser)”, Statens Kartverk.
Norwegian Ministry of Climate and Environment (2013), “Climate change adaptation in Norway`- Meld. St. 33 (2012–2013)”, Report to the Storting (white paper), available at: https://www.regjeringen.no/en/dokumenter/meld.-st.-33-20122013/id725930 (accessed 6 March 2022).
Norwegian Water Resources and Energy Directorate (NVE) (2014), “Floods and landslides in land use plan”, Oslo, available at: https://publikasjoner.nve.no/retningslinjer/2011/retningslinjer2011_02.pdf (accessed 26 January 2023).
Norwegian Water Resources and Energy Directorate (NVE) (2016a), “Climate change and future floods in Norway”, Oslo, available at: https://publikasjoner.nve.no/rapport/2016/rapport2016_81.pdf (accessed 26 January 2023).
Norwegian Water Resources and Energy Directorate (NVE) (2011), “NVE’s strategy for climate change adaptation 2015-2019”, Oslo, available at: https://www.nve.no/ (accessed 6 March 2022).
Norwegian Water Resources and Energy Directorate (NVE) (2016b), “Preliminary flood risk assessment in Norway. An example of a methodology based on a GIS-approach”, Oslo, available at: https://publikasjoner.nve.no/report/2011/report2011_07.pdf (accessed 23 August 2022).
Olstad, T.M. (1994), “Mediaeval wooden churches in a cold climate—parish churches or museums?”, Studies in Conservation, Vol. 39, pp. 99-103.
Olstad, T.M. (2016), “To the glory of God and the church’s adornment”, in Bakken, K. (Ed.), Preserving the Stave Churches. Craftmanship and Research, Pax Forlag, Riksantikvaren.
Olstad, T.M. and Ørnhøi, A.A. (2014), “Konsolidert Limfargedekor I Stavkirkene-EnOversikt. Vurdering Av Størlim. Konsolidering Av Limfarge På Tre.Delprosjekt 1”, NIKU Oppdragsrapport 62/2014, Norsk Institutt Forkulturminneforskning (NIKU), Oslo.
Olstad, T.M. and Solberg, K. (1998), “Eight seventeenth-century decorative paintings-one painter?”, Studies in Conservation, Vol. 43, pp. 175-179.
Olstad, T.M., Ørnhøi, A.A., Jernæs, N.K., de Ferri, L., Freeman, A. and Bertolin, C. (2020), “Preservation of distemper painting: indoor monitoring tools for risk assessment and decision making in Kvernes stave church”, Climate, Vol. 8 No. 2, p. 33.
Ortiz, R., Ortiz, P., Martín, J.M. and Vázquez, M.A. (2016), “A new approach to the assessment of flooding and dampness hazards in cultural heritage, applied to the historic centre of Seville (Spain)”, Science of the Total Environment, Vols 551-552, pp. 546-555, doi: 10.1016/j.scitotenv.2016.01.207.
Paille, M., Reams, M., Argote, J., Lam, N.S.-N. and Kirby, R. (2016), “Influences on adaptive planning to reduce flood risks among parishes in south Louisiana”, Water (Basel), Vol. 8 No. 2, doi: 10.3390/w8020057.
Park, S., Choi, C., Kim, B. and Kim, J. (2013), “Landslide susceptibility mapping using frequency ratio, analytic hierarchy process, logistic regression, and artificial neural network methods at the Inje area, Korea”, Environmental Earth Sciences, Vol. 68, pp. 1443-1464.
Pascale, S., Pastore, V., Sdao, F. and Sole, A. (2013), “Landslide susceptibility in archaeological and natural historic Park of rupestrian churches”, in Margottini, C., Canuti, P. and Sassa, K. (Eds), Landslide Science and Practice, Vol. 6, pp. 715-722, doi: 10.1007/978-3-642-31319-6_63.
Paupério, E., Romão, X. and Costa, A. (2012), “A methodology for the vulnerability assessment of heritage buildings and contents under catastrophic hazards. ICOMOS-ICORP”, International symposium on cultural heritage protection in times of risk: challenges and opportunities, Istanbul.
Previtali, M., Stanga, C., Molnar, T., Meerbeek, L. and Barazzetti, L. (2018), “An integrated approach for threat assessment and damage identification on built heritage in climate-sensitive territories: the Albenga case study (San Clemente church)”, Applied Geomatics, Vol. 10, pp. 485-499, doi: 10.1007/s12518-018-0217-3.
Sabbioni, C., Brimblecombe, P. and Cassar, M. (2010), The Atlas of Climate Change Impact on European Cultural Heritage. Scientific Analysis and Management Strategies, Anthem Press, London, New York, Melbourne, Delhi.
Sesana, E., Gagnon, A.S., Ciantelli, C., Cassar, J.A. and Hughes, J.J. (2021), “Climate change impacts on cultural heritage: a literature review”, WIREs Climate Change, Vol. 12 No. 4, e710, doi: 10.1002/wcc.710.
Sevieri, G., Galasso, C., D’Ayala, D., De Jesus, R., Oreta, A., Grio, M.E.D.A. and Ibabao, R. (2020), “A multi-hazard risk prioritisation framework for cultural heritage assets”, Natural Hazards and Earth System Sciences, Vol. 20, pp. 1391-1414, doi: 10.5194/nhess-20-1391-2020.
Solberg, K., Norsted, T. and Spaarschuh, C. (2007), “Eidsborg Stavkirke. Konservering Av Veggmalerier Fra 1600-Tallet Og Paneler Fra Middelalderen No. 35/2007”, Norsk Institutt for Kulturminneforskning (NIKU), Oslo.
Stephenson, V. and D`Ayala, D. (2014), “A new approach to flood vulnerability assessment for historic buildings in England”, Natural Hazards and Earth System Sciences, Vol. 14, pp. 1035-1048.
Tyagunov, S., Heneka, P., Stempniewski, L., Zschau, J., Ruck, B. and Kottmeier, C. (2005), “CEDIM: from multi-hazards to multi-risks”, Proceedings of the 1st ARMONIA conference.
UNESCO (2008), “Policy document on the impacts of climate change on world heritage properties, document WHC-07/16. GA/10 adopted by the 16th GeneralAssembly of states Parties to the world heritage convention (October 2007)”, World Heritage Center UNESCO, Paris, France, p. 32, available at: https://whc.unesco.org/document/10046 (accessed 26 January 2023).
UNISDR (United Nations International Strategy for Disaster Reduction) (2005), Hyogo Framework for Action 2005–2015: Building the Resilience of Nations and Communities to Disasters, UNISDR, Geneva.
UNISDR (United Nations International Strategy for Disaster Reduction) (2015), Sendai Framework for Disaster Risk Reduction 2015–2030, UNISDR, Geneva.
United Nations Office for Disaster Risk Reduction (2015), Sendai Framework for Disaster Risk Reduction 2015-2030, United Nations International Strategy for Disaster Reduction, Geneva, available at: https://www.undrr.org/publication/sendai-framework-disaster-risk-reduction-2015-2030
Wedvik, B.A. (2008), “Heddal Stavkirke. Konsolidering Av Limfargedekor I Koret. Prosjektets Del 1: Nordvegg Og Apsis I Koret No. 49/2008”, Norsk Institutt for Kulturminneforskning (NIKU), Oslo.
Wedvik, B.A. (2009), “Heddal Stavkirke. Konsolidering Av Limfargedekor I Skip Og Sørvegg I Koret No. 121/2009”, Norsk Institutt for Kulturminneforskning (NIKU), Oslo.
Wilson, D., Fleig, A.K., Lawrence, D., Hisdal, H., Pettersson, L.E. and Holmqvist, E. (2011), “Report no. 9 – 2011. A review of NVE’s flood frequency estimation procedures”, Norwegian Water Resources and Energy Directorate, Oslo.
Youssef, A.M., Pradhan, B., Jebur, M.N. and El-Harbi, H.M. (2015), “Landslide susceptibility mapping using ensemble bivariate and multivariate statistical models in Fayfa area, Saudi Arabia”, Environmental Earth Sciences, Vol. 73, pp. 3745-3761.
Acknowledgements
This work was supported by the Sustainable Management of Heritage Buildings in a Long-term perspective (SyMBoL) Project (Project No. 274749). The second author is grateful to the Norwegian University of Science and Technology (NTNU), who hosted her as a visiting researcher for the academic collaboration.