Abstract
Purpose
This paper aims to present the application of a tailored systematic engineering design procedure to the concept design of a small production plant for compostable packaging made by straw fibres and bioplastic. In particular, the obtained boxes are intended to be used for wine bottles.
Design/methodology/approach
A systematic procedure has been adopted, which underpins on a comprehensive analysis of the design requirements and the function modelling of the process. By considering well-known models of the engineering design process, the work focuses on the early design stages that precede the embodiment design of the whole components of the plant.
Findings
The followed design approach allowed to preliminarily evaluate different alternatives of the process from a functional point of view, thus allowing to identify the preferred conceptual process solution. Based on the identified functional sequence, a first evaluation of the potential productivity and the required human resources has been performed.
Research limitations/implications
The procedure shown in this work has been applied only for the considered case of compostable packaging, and other applications are needed to optimize it. Nevertheless, the adopted systematic approach can be adapted for any context where it is necessary to conceive a new production plant for artefacts made by innovative materials.
Originality/value
The work presented in this paper represents one of the few practical examples available in the literature where systematic conceptual design procedures are presented. More specifically, to the best of the authors’ knowledge, this is the very first application of systematic design methods to compostable packaging production.
Keywords
Citation
Fiorineschi, L., Conti, L., Rossi, G. and Rotini, F. (2022), "Conceptual design of a small production plant for eco-friendly packaging", Journal of Engineering, Design and Technology, Vol. ahead-of-print No. ahead-of-print. https://doi.org/10.1108/JEDT-02-2022-0118
Publisher
:Emerald Publishing Limited
Copyright © 2022, Lorenzo Fiorineschi, Leonardo Conti, Giuseppe Rossi and Federico Rotini.
License
Published by Emerald Publishing Limited. This article is published under the Creative Commons Attribution (CC BY 4.0) licence. Anyone may reproduce, distribute, translate and create derivative works of this article (for both commercial and non-commercial purposes), subject to full attribution to the original publication and authors. The full terms of this licence may be seen at http://creativecommons.org/licences/by/4.0/legalcode
1. Introduction
Traditional packaging obtained from fossil sources (e.g. polystyrene and its derivate) still constitutes the first choice for industry, despite the number of concerns about the negative impact on environment (Davis and Song, 2006; Tencati et al., 2016). In particular, the landfill treatment of packaging waste and persistence in the environment need urgent and ecological solutions (Ferdous et al., 2021; Nordin and Selke, 2010; Petersen et al., 1999; Weale et al., 2010). More precisely, packaging is responsible for a third of all plastic produced worldwide, and in industrialized western countries, half of the goods sold on the market have a plastic packaging (E.V., 2018). For these reasons, the problem of plastic packaging is particularly critical in emerging economies (Pani and Pathak, 2021). However, even if limiting to the European Union, 70 million tons of packaging waste have been produced in 2016, approximately 16.7 million tons of which were made of plastic (Dahlbo et al., 2018; Tallentire and Steubing, 2020).
Perhaps for these reasons, packaging is often considered one of the most critical elements for making innovation and improvements in different supply chains (García-Arca et al., 2017, 2020). Accordingly, hi-tech sustainable solutions have been recently investigated (Moustafa et al., 2021, 2022), but also the development of packaging materials deriving from renewable resources, such as organic waste, has received increasing attention. Indeed, and especially for the industrial sector, that of packaging is now widely acknowledged as a pressing need (Babalis et al., 2013). Unlike synthetic polymers, biodegradable polymers such as cellulose, polysaccharides, proteins, from crops or biomass, can undergo physical, chemical or biological decomposition, being suitable for inclusion in composting processes at both domestic and industrial scales (Meeks et al., 2015). This kind of materials would open up new opportunities for recycling waste, which are more advantageous than landfill, both in environmental and in economic terms (Garrido et al., 2014). In particular, the possibility of using agricultural waste has gained increasing attention in literature (De Pilli et al., 2021). For example, due to the large production scales, the cereal industry can be an optimal source of natural fibres to be used in biodegradable polymers since the related waste (the straw), besides the animal nutrition, is particularly suitable for the manufacturing of compostable products. Accordingly, the overall amount of straw produced each year from the cereal industry is about 2.1 billion tons.
In this context, a particular type of compostable material has been recently proposed (Rossi et al., 2020) and will be taken as a reference in this work. The material (NeoPalea) is a compressed composite made by straw and bioplastic (Mater-bi). By definition, bioplastics are made of natural polymers (polysaccharides such as starch and cellulose) extracted from renewable agricultural resources (corn, potato, tapioca, rice, sugar cane, etc.) as well as ligno-cellulosic fibres (European Committee for Standardization, 2010).
The considered composite, however, has been manufactured only in a laboratory-scale to perform the tests for manufacturability, to extract information about the mechanical characteristics and to evaluate the actual composting properties of the material. Therefore, industrial manufacturing procedures for obtaining packaging with this material are currently missing. This is acknowledged to be an obstacle for the industry since the uncertainties behind newer packaging technology may make it hard to design sustainable packaging processes (Ma and Moultrie, 2018).
A first application of the material has been found and consists in the realization of the packaging for wine bottles in a farm production scale, with full availability of straw and limited human resources. Indeed, current eco-friendly wine cases for bottles are usually manufactured with wood panels and bottles are protected with straw tufts. However, some recycling issues may arise with this kind of product. For example, the use of metallic nails limits the composability, and the rigid structure of the case needs to be dismounted to save space before use. Accordingly, to support the environmental sustainability of wooden cases for wine bottles, disassembly protocols have been proposed in literature (González-García et al., 2011b) as well as the reuse of related parts in do-it-yourself activities (González-García et al., 2011a). Differently, the NeoPalea allows to obtain the form of packaging parts, which could be assembled without the use of nails of glues. Additionally, it does not need to be disassembled at the end of the life, but it can be directly placed in domestic composters. It is also important to mention that the wine market is quite wide, including very different classes of wine, from low-cost to expensive wines. While it has been found that the bag-in-box solution can actually be considered as one of the most sustainable solutions (Ferrara and De Feo, 2020), it can be exploited only for low-cost wines. More expensive classes of wine are usually sold with glass bottles specifically dedicated and related packaging. The use of glass is also justified by the superior properties that it shows in terms of flavour preservation (Thompson-Witrick et al., 2021). Accordingly, the wine case considered in this work is expected to be used for medium-high classes of wines, which actually need to be sold into glass bottles. Additionally, the use of biodegradable packaging has been recently found as an attractive feature from consumers (Cammarelle et al., 2021).
The extension of the plant for the production of NeoPalea wine cases is expected to fit in 150 square meters, with a production of about 60 bottle’s casings per day. The expected application and location also underpin the motivations that led towards this specific compostable material. Indeed, the plant to be designed is intended to be located in a farm with a wide availability of straw fibres. The first attempt to obtain a sustainable packaging for this specific application has been then guided by the amount of available organic fibres.
The aim of this study is to perform the conceptual design of a production process for wine bottle casings made by the considered ecological composite. Accordingly, it is important to put beforehand that nothing concerning the subsequent design phases (i.e. the embodiment and the detail design phases) is addressed here. A systematic design process has been developed, inspired to the well-known Pahl and Beitz engineering design textbook (Pahl et al., 2007), integrated with the use of a recently proposed tool for enhancing the extraction of design requirements (Fiorineschi et al., 2020a). Indeed, the challenge of sustainability requires systematic approaches to design solutions capable to fulfill the stakeholders’ needs and to minimize the resources’ consumption. In such a perspective, a functional design of the process is then performed, and a schematic layout of the plant is realized to perform preliminary evaluations about the actual production issues and the number of operators required. Furthermore, the design case allowed to check the applicability of the developed systematic approach to the considered problem.
The followed methodological approach is comprehensively explained in Section 2, together with a description of the considered composite material. The obtained results, i.e. the conceptual design outcomes, are described in Section 3. Discussions are reported in Section 4, where the limits of the works are clearly highlighted, and the expected impact is discussed. Eventually, formal conclusions are reported in Section 5. Eventually, in the Appendix section, the complete requirement list of the production process is made available to the reader.
2. Materials and methods
2.1 The eco-friendly packaging material
The eco-friendly composite material used in this work is composed by straw and bio-plastic (Rossi et al., 2020), whose capability to be used for packaging applications has been tested to a laboratory scale. In particular, several shapes have been obtained, which constitute hypothetical parts of generic packaging goods (samples are shown in Figure 1).
To obtain the samples, a manual process has been developed to prepare the raw material. The main phases are reported in the following:
Rolling straw into a bio-plastic sheet to obtain cylinders of about 20 cm length with a diameter of approximately 3–4 cm (see Figure 2).
Deposition of the required number of cylinders into the mould.
Pressing the material into the mould.
Heating the mould.
Cooling the mould.
Extracting the obtained product from the mould.
The hypothesized packaging to be produced should be made of two equal parts, each of them constituting the exact half of the packaging (Figure 3). The form shown in Figure 3 is just a concept to give an idea of the main dimensions, while the details will be studied in subsequent design steps.
2.2 Conceptual design of the manufacturing process
Some functional parts of the production process can be directly extracted from the lab-scale experiences, but many other functions are required for the implementation of the small production plant. Moreover, the technical systems for some of the key functions are not available in the market. It implies that it is not possible to proceed directly with an embodiment design of the plant. Indeed, it is necessary to carefully identify all the necessary functions and to discuss about the feasibility of the project from an abstract point of view. This is fundamental to allow the extraction of the specific design requirements for the development of the new technologies needed for implementing the key functions that cannot be fulfilled with standard devices (for example, the preparation of the composite raw material). Additionally, the outcomes of the conceptual design of the plant are fundamental to identify suitable devices for the other functions.
By following well-known systematic engineering design procedures (Pahl et al., 2007), the first step of any design process consists in clarifying the design task. Accordingly, also in the present work, this step is fundamental to provide the information required for planning an effective conceptual design of the production process. By referring to the schematization shown in Figure 4, Steps 1 and 2 implement the most important task clarification activities, i.e. the identification of the main functions (Step 1) and the identification of the design requirements (Step 2).
The activities related to Steps 1 and 2 in Figure 4 have been performed by referring to the past experiences gathered by some of the authors when performing preliminary laboratory tests on the considered composite material. More precisely, the hand-conducted process currently used to prepare the cylinders constitutes the base for extracting the main functions characterizing the process to the industrial scale. Then, for each of these main functions, the requirement checklist of Pahl and Beitz (Pahl et al., 2007) has been used to elicit the information for different categories (Step 2 in Figure 4). More, in particular, the considered process took advantage from a recently proposed matrix-based procedure, which helps the designer in managing the requirements for the different functions (Fiorineschi et al., 2020a). Indeed, the latter approach allows to exploit any requirements checklist and to link each requirement category to each main function identified for the system or the process to be designed (Fiorineschi et al., 2020a).
To limit the negative effects of design fixation (Jansson and Smith, 1991; Purcell and Gero, 1996), the design space explorations for this conceptual design session has been performed at an abstract level, i.e. by relying on functional models. In particular, functions are considered here as actions performed by the technical system (the manufacturing process), capable of modifying fluxes of energy, material and/or signal (Pahl et al., 2007), known as the Energy-Material-Signal (EMS) model (see Figure 5).
However, since the elements implementing some functions are still not available, it is not possible to determine the energy fluxes a-priori, as well as most of the signal-related fluxes (that are heavily influenced by the solution to be determined). Therefore, only material fluxes are considered in this work, thus allowing to model each main function of the system and to put it in a logical and procedural sequence (Step 3 in Figure 4). In this way, different process variants have been proposed and analysed, in an iterative fashion, by means of interactions among the designers (the two authors from the Engineering Department), the scientists (the two authors from the Agricultural Department) and the potential final user (the owner of the farm). Thanks to this abstract modelling process, it has been possible to compare and evaluate different strategies and hypothesize different solutions (Step 4 in Figure 4). More precisely, for each function, it has been possible to search current available technologies that could be potentially suitable for an implementation. Then, by gathering information from suppliers and/or manufacturers of such technologies (e.g. costs, power consumption and applicability for the specific material), it has been possible to take decisions. Where it was not possible to find already existent technologies, the estimate was based only on shared discussions performed by the authors of this work. In particular, the engineering team proposed and evaluated potential solutions (in an abstract form), according to the requirements expressed by the agroforestry research team (i.e. concerning the manufacturing process of the pseudo-cylinders and the forming procedures). The two teams jointly performed evaluations of the different alternatives and selected the preferred ones.
To perform the estimates about the potential productivity and the required human resources, a schematic representation of the plant is used to visualize the required stations (Step 5 in Figure 4). Contextually, the number of required human resources is evaluated by identifying the manually operated stations and by optimizing the process in terms of minimum number of human operators required, according to the expected productivity and the planned budget. In particular, the time required for each operation (according to the developed functional model and its schematization as a plant) has been preliminary estimated by considering highly precautionary time intervals (Step 6 in Figure 4). It is then expected that the final process would be optimized in further design iterations (not faced in this work), thus leading to an even more productive plant.
3. Results
3.1 Design requirements and main functions
By exploiting the tool proposed by Fiorineschi et al. (Fiorineschi et al., 2020a), it has been possible to extract a detailed set of design requirements characterizing this specific task. In particular, by relying on the requirements checklist of Pahl and Beitz (specifically tailored for conceptual design activities), objectives and constraints have been identified, expected for each main function of the system, together with transversal requirements valid for the whole plant.
More specifically, through a first analysis of the manual process used in laboratory, together with an interview session performed with the academic staff and the potential user, it has been possible to obtain a list of the main functions that characterize the process to be designed (see Table 1).
The complete lists of obtained requirements, expressed in the forms of objectives and constraints is reported in Appendix and has been used as a reference for the whole process. However, due to the iterative nature of the design process, it is important to highlight that the mentioned set of requirements constitutes the starting point of the design process and the list will be updated and upgraded when needed (e.g. when major details of the investigated solutions appeared) (Roozenburg and Eekels, 1991).
3.2 Preferred functional model of the process
The whole preferred functional model of the manufacturing process is represented in Figure 6, which has been subdivided into three parts (A, B and C) for visualization purposes. Indeed, a magnified version of each of the three parts is shown in Figures 7, 8 and 9. The part “A” (Figure 7) represents the set of functions that starts from the import of both fibres and bioplastic up to the transportation of the manufactured pseudo-cylinders to the mould preparation site.
The part “B” (Figure 8) contains the set of functions devoted to the preparation of the mould, which is performed by means of a process carried out mainly by hand. Then, in the part “C” (Figure 9), the set of functions are shown, which are devoted to the realization and the extraction of the final packaging product. In the following paragraphs, each part is described in detail.
In particular, Figure 7 shows the sequence of functions needed to transform straw fibres and two layers of bioplastic into an array of pseudo-cylinders. First of all, the straw fibres are extracted from a straw bale and aligned to be placed within two layers of Mater-bi. Then, this “sandwich” bioplastic-straw-bioplastic is processed by a thermo-soldering function aimed at obtaining the array of pseudo-cylinders. Since the desired length of the pseudo-cylinders is known, a transversal cut is then performed to obtain the final “chains” of composite material (see the sketch in Figure 7) to be moved to the subsequent process station.
Figure 8 shows the sequence of functions related to the preparation of the mould. More, in particular, a conventional “release agent” is deposed into the mould to ease the extraction of the packaging after the forming process. The release agent is meant to be fully bio-compatible and degradable. Products from the food industry have been taken into consideration for this specific function (see, for example, www.dubor.de).
After the deposition of the release agent, foils of Mater-bi are placed both into the mould and on the lid. Then, the required number of pseudo-cylinders are placed into the mould, and the lid is placed over the mould.
Figure 9 shows the last set of functions, and in particular, the main forming functions are reported. The mould (previously prepared with the required material) is compressed and heated to the required temperature, and locked in position to maintain the compression after the removal from the press. This characteristic is fundamental to allow the full exploitation of a single press and then to improve the related productivity. Indeed, without the locking function of the moulds, the press would be maintained in pressure with the same set of moulds for all the required forming time.
The mould is then transported to the cooling station to speed up the cooling time. Once the mould reaches the room temperature, it can be unlocked and the packaging extracted. The empty mould and the related lid are then ready to be washed and reused for another cycle.
3.3 Schematic representation of the plant
By considering the functional model of the process shown in Figures 6 to 9, it has been possible to conceive a schematic layout of the plant. In particular, the “stations” required to implement the process functions have been represented in Figure 10, together with the forklift operations that are needed to move heavy loads. A short description of each station and forklift operations are reported, respectively, in Tables 2 and 3.
3.4 Estimate of the required human resources
The key function and the related station considered as the bottleneck of the process, is the number 9 (heating press) in Figure 10. More specifically, the heating press station has been considered as the most time-consuming among the “active” operations. Indeed, “passive” operations like the storage or the mould cooling do not have any impact to the rest of the process (unless the required storage space is compatible with the available building). For the heating press, a specific technology has been considered, i.e. an hydraulic press with board heated by means of diathermic oil. It is possible to find many manufacturers for this acknowledged technology. More, in particular, a press with a standard working plane with dimensions of 3,000 mm length and 1,300 mm wide has been considered (cost of about €35.000).
By considering the hypothesized dimensions of the semi-package (see Figure 3), it is possible to imagine the complete mould (assembly composed by the mould and the lid made in aluminum) as shown in Figure 11.
The hypothesized form for the mould (Figure 11) allows to consider the possibility to move a set of 18 moulds with the forklift by means of a specific loading rack to be mounted in place of the standard forks (see Figure 12). In this way, by considering the dimensions of the working plane of the press, a complete load can be reached with two passages of the forklift.
With this information, and by hypothesizing the time required for the other operations shown in Figure 10, it has been possible to perform a first rough verification about the potentialities of the plant (even if not designed at an embodiment stage). More specifically, the chronological sequences of the operations have been established, especially for human-operated stations (Figure 13). As a result, it has been found that with the hypothesized operation times, for a complete process (from Steps 1 to 15 in Figure 10), an estimated time of 260 min is required.
A standard working day is composed by 8 h, i.e. 480 min. Therefore, if considering a sequential logic (i.e. a new process starts only when the precedent one ends), it is not possible to complete two cycles. In other words, by considering the hypothesized moulds and the heating press dimensions, more than a working day is needed to obtain only 72 semi-packages, i.e. 32 wine cases. This is not compatible with the initial requirement of 60 wine cases per day.
Nevertheless, it is possible to organize the process to start more cycles on the same day and then for obtaining larger production volumes. To do that, it is fundamental to carefully consider the operation times hypothesized for each station and for the forklift (see Figure 13). In particular, for the forklift and for the manually operated stations, it is fundamental to consider the actual number of available operators. Indeed, two different manually operated stations cannot work if there are not available humans to manage them.
The reference functions considered for estimating the sequences are those related to the heating-press (Steps 9 in Figures 10 and 13). Indeed, all the process works around the productivity of the press. Accordingly, two forklift operations (load and unload) have been considered, together with the 60 min required for pressing and heating the moulds. By referring to precedent laboratory trials, the time required for the cooling has been estimated to be 120 min. However, once the moulds have been moved to the cooling station, the press can be re-loaded with the other 36 moulds.
By considering this simple fact, it has been possible to estimate the maximum productivity reachable with a single operator and a forklift operator (total of two operators in the whole plant).
More precisely, according to the approach described in Section 2, by referring to both the functional model of the process (Figures 4 to 7) and the schematic representation of the plant (Figure 10), it has been possible to identify the minimum number of human operators, which is deemed sufficient to obtain the required productivity. As shown in Figure 14, it has been possible to organize four sequential cycles per day (i.e. 72 wine cases per day), according to the process sequence and the considered human resources. Indeed, the production estimate has been performed by considering that a manually operated station can work only if the operator is available. Additionally, the process sequence has been preserved (e.g. Station 6 in Figure 10 cannot work if Station 4 has not produced the required pseudo-cylinders).
4. Discussions
4.1 Achieved results
The followed systematic conceptual design procedure allowed to identify a comprehensive set of design requirements and to clearly highlight the main functions of the process. By starting from this initial set of information, a preferred functional model has been developed and used for conceiving a first concept of a hypothetical plant capable to comply with the design requirements. Some of the identified functions can be implemented with already available technologies, e.g. for the pressing and heating of the mould. Since these two functions can be implemented by a single device (the heating press), a potentially suitable device has been identified in the market and has been used as a reference for developing the process. Indeed, the heating and pressing functions constitute the bottleneck of the process since only one device can be used (according to the available budget), and the time required for the functions is relatively high. Other secondary (but important) functions have been identified, such as the release agent application or the washing of the empty moulds and related lids. These two functions are fundamental to limit the number of moulds by re-using them in subsequent process cycles. In particular, the release agent allows to easily extract the semi-package and then to reduce the residuals to be removed by the washing station.
However, one of the most critical functions is the production of the pseudo-cylinders, but no available technology can be used in this case. Therefore, it has been assumed that the new device to be designed would be capable to provide the required productivity, and the required human resources have been hypothesized. These assumptions are the first set of specifications for the new technology to be designed.
Thanks to the schematized process reported in Figure 10, it has been possible to identify the required forklift operations and the related need of human resources.
As a result, estimates about the productivity and the number of the required operators have been performed. Therefore, even by considering precautionary values for the time required in each operation, the hypothesized process seems to comply with the required minimum production by using only two human operators. In particular, to perform such an estimate, it was crucial to hypothesize a first rough form for the mould, to understand how many of them could be processed by the heating press for each pressing cycle.
4.2 Limits and future developments
The estimates performed in this work are based on assumptions made on abstract solutions identified for implementing the functions characterizing the whole process. One of these assumptions concerns the form of the mould and the possibility to “lock” the related lid once compressed under the press. This assumption is fundamental for the success of the hypothesized process since it allows to sensibly reduce the operating time of the heating press. Some hypothetical solutions have been identified for the locking mechanism, which of course, should be capable to be easily unlocked. However, until further embodiment details are developed and tested, it is not possible to perform comprehensive estimated about the time required for extracting the product from the cold moulds.
Additionally, also the estimate about the time needed for the cooling of the moulds should be performed only when the actual geometry has been decided. Nevertheless, for this specific scale of the process, the cooling time appears to be not so critical due to the availability of spaces where to place the hot moulds. Differently, with higher production rates, the cooling of the moulds could be extremely crucial because of the high volumes required to stock them. In these cases, a mould with cooling channels (Hussain and Safiulla, 2018; Venkatesh et al., 2017) could be considered, while is deemed too expensive and not justified for the production scale considered in this work.
Concerning the realization of the pseudo-cylinders, the main limitation concerns the actual absence of any real device to be considered for performing comprehensive estimates and verifications. The assumption that a new technology can be capable to successfully implement the function is quite strong, and cannot be validated here. However, preliminary tests performed in the laboratory showed that the Mater-bi foils can be easily soldered at relatively low temperatures (Fiorineschi et al., 2021a). It allowed to imagine potentially feasible concepts of the device, operating on pre-aligned straw fibres placed within two layers of Mater-bi (coming from two distinct rolls). This idea allows thinking about a continuously-operating device where the two layers of bioplastic and the pre-aligned fibres constitute the inputs, and the pseudo-cylinders are obtained by a heated roll and a transversal cutter. In this way, “arrays” of pseudo cylinders are expected to be produced. The design of the device constitutes the very next step of this work and is based on the hints and assumptions provided here.
Concerning the biodegradability of the packaging, some tests should be performed on the final geometry to verify if the performance observed in for the early vermicomposting tests performed by Rossi et al. (2020) on the Neopalea specimens can be confirmed.
There is also the possibility to adapt the results obtained in this work to obtain other packaging products as the needed functions are invariant and fully scalable with respect to the shape and dimensions of the packaging. These outcomes have been achieved through the systematic design approach that has been adopted, which allows to organize the cognitive process in different levels, from the abstract (i.e. design of the process functions) to the detailed one (technologies to implement the functions). More specifically, the concept design presented here is best suited for cases of limited dimensions (comparable with the considered wine case) and for limited production rates. Indeed, the design requirements that underpin this work led to a tailored concept of the plant based on the NeoPalea material. Nevertheless, the methodological approach can be applied to other cases, without any limit of material, forms or dimensions of the packaging.
Another limitation of the work concerns the absence of comprehensive comparisons with production processes about current wood-based wine cases. Such an activity should be performed as soon as technologies and related devices have been identified or designed for each functional station of the plant.
Eventually, only straw fibres have been considered in this work, while it is possible to evaluate the possibility of using different biological raw materials, as, for example, leafs, which have been already used in other contexts for the production of bricks (Sahu et al., 2020).
4.3 Expected impact
This work, despite the limitation listed in the previous paragraphs, demonstrates that sustainable processes can be designed for the production of ecological packaging made by natural fibres and bioplastic. From the societal point of view, this is extremely important because it allows people to understand that plastic can be actually eliminated or drastically reduced in the packaging industry, at least for some products.
The impact for academia is twofold. Indeed, this paper shows that a laboratory-scale manufacturing process developed for the production of the composite can be transformed into a real production process by following systematic steps. Then, the method adopted in this work and explained in this paper provides a reference for scholars involved in similar activities that need to convert a laboratory-scale process into a concept of a real plant. The other impact expected for academia is expected for scholars involved in the Design Science. Indeed, this work shows a particular application of methodological tools and design models that actually bridges (for this specific application field) the gap that exists between academia and industry when talking about design methods. Indeed, the methodological approach developed in this work has been thought to bring insightful ideas from academia towards realistic industrial application. Additionally, the work shown in this paper is one of the few actually available in literature, where it is possible to observe a practical application of systematic conceptual design approaches derived from academia (Fiorineschi et al., 2021b, Fiorineschi et al., 2020b).
Finally, also many impacts for the industry are, of course, expected. Some of them can be easily deduced by the societal and academic impact introduced above, where the industry is somewhat involved. Additionally, the methodological approach shown in this paper can be successfully used by industrial practitioners interested in evaluating new potential applications coming from academia. Indeed, notwithstanding the specific application of the method that has been shown in this paper, similar approaches can be used to rapidly transform a lab-scale process into comprehensive conceptual estimates of potential industrial processes. Nevertheless, the main impact for industry is the possibility to refer to this work as a real opportunity for a new sustainable product that can actually reduce the ecological impact of packaging.
5. Conclusions
The work described in this paper is aimed at proposing a concept for a sustainable production plant for compostable packaging. In particular, the considered material was composed by the Mater-bi bioplastic and straw fibres, while the packings were intended as cases for wine bottles.
A small production volume (60 wine cases per day) has been considered, according to the wine bottle production for a reference Italian farm. To perform the conceptual design process, the authors took inspiration from well-known systematic procedures normally used in the engineering field. More specifically, a tailored conceptual design process has been developed, where the main key steps are focused on the extraction of a comprehensive list of design requirements, and in-depth functional modelling. Thanks to the adopted approach, it has been possible to conceive a schematic layout of the plant and to perform first estimates about the potential productivity with a minimum number of human operators (according to the available project budget).
The limitations of this work consist in the subjectivity of the assumptions made to overcome the current absence of suitable technologies for implementing some of the functions of the process. Nevertheless, the assumptions have been performed in a precautionary way by a series of discussions among the authors (from both engineering and agroforestry departments).
The obtained results pave the way for the design of the missing technologies and for the future embodiment design of the whole plant. Additionally, the systematic approach used in this work can be conveniently reused by scholars and/or industrial practitioners involved in the development or the analysis of potential production processes for innovative materials.
Figures
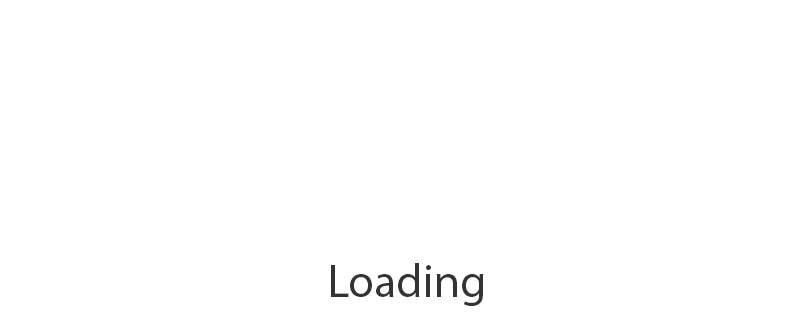
Figure 7.
Detailed view of the “A” part of the functional model shown in Figure 6
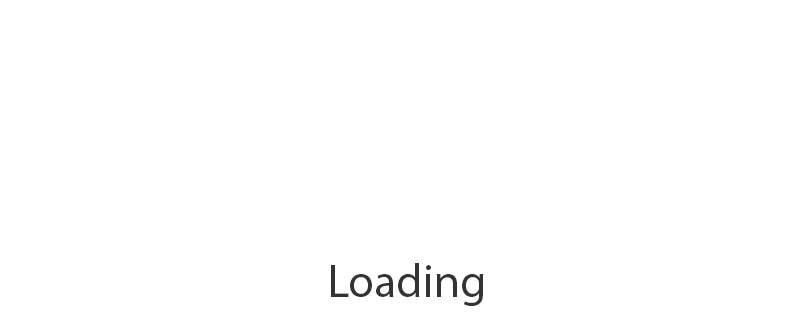
Figure 8.
Detailed view of the “B” part of the functional model shown in Figure 6
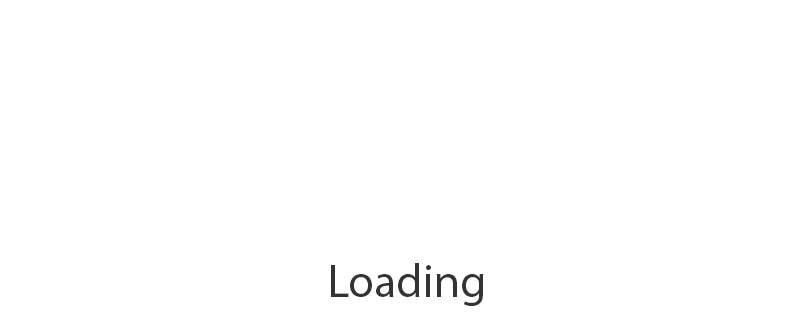
Figure 9.
Detailed view of the “C” part of the functional model shown in Figure 6
Main functions expected for the plant to be designed
Function | Description |
---|---|
Import bales | The system is expected to work with straw bales. This specific function represents the need to import the bales into the plant |
Unroll bales | This specific function represents the need to obtain straw fibres from bales |
Cut straw fibres | Since the length of the required straw-bioplastic cylinders is generally inferior to the normal length of straw fibres, it is necessary to consider the need to cut the fibres |
Import Mater-bi | The system is expected to work with bioplastic films. This specific function represents the need to import this material into the process |
Roll bio plastic + straw | This is the most important function, which is expected to transform straw fibres and bio-plastic films in the desired cylinders |
Placing the cylinders into the mould | This function is expected to be performed manually. Therefore, it is not investigated in this work |
Compress cylinders into the mould | This function represents the need to generate a compression force and to apply it on the mould to shape the cylinders into the desired form |
Heating the mould | This is a fundamental function needed to obtain the composite material |
Cooling the mould | The obtained form cannot be extracted until the mould is at room temperature |
Extract the semi-package | The extraction of the form is performed manually |
Export the semi-package | This function represents the need to extract the obtained form from the plant |
Description of the stations shown in Figure 10
Station | Description | Operation groups |
---|---|---|
1 | Bales storing zone. This is a zone where the straw bales required for the process are stored | Pre-press operations |
2 | Bale dissolver station. In this station, a bale dissolver device is installed to extract straw fibres | |
3 | Fibres alignment station. For this specific scale of the process, the alignment of the fibres is manually performed by a human operator | |
4 | Pseudo-cylinders forming station. A specific device (which still needs to be designed) is intended to be used for joining Mater-bi and straw fibres into the form of pseudo-cylinders | |
5 | Mater-bi storing zone. This is a zone where the bioplastic rolls required for the process are stored | |
6 | Pseudo-cylinders sorting station. In this station, an operator sorts the arrays of pseudo-cylinders in the number and the forms (i.e. length of the “chain” of pseudo-cylinders) required to fill the mould | |
7 | Mould preparation station. A human operator deposes the precedently prepared pseudo-cylinders into the mould | |
8 | Moulds loading station. The moulds are loaded into a specific rack, which can be easily moved by a forklift towards the heating press | |
9 | Pressing and heating station. The loaded heating press starts the cycle, and mantain the temperature for the required time | Pressing operations |
10 | Moulds cooling station. Once extracted from the heating press, the moulds are placed into a cooling rack until the room temperature is reached | Cooling operations |
11 | Semi-packaging extraction station. The mould is opened, and the formed packaging is extracted. This operation is performed manually by the help of possible tools specifically designed to ease the operation | Post-cooling operations |
12 | Semi-packaging storing zone. In this zone, the finished semi-packaging are stored | |
13 | Empty moulds washing station. This is a manually operated station where the operator uses pressurized water to remove possible residuals from the mould | |
14 | Empty moulds storage zone. In this zone, the washed empty moulds are stored, waiting for the next cycle | |
15 | Release agent application station. This is a manually operated station, where an natural release agent (for food applications) is sprayed on both the mould and the lid internal surfaces |
Description of the forklift operations characterizing the plant shown in Figure 10
Forklift operation | Description |
---|---|
A | Transporting the bales from the storage to the dissolver |
B | Press loading |
C | Extraction of the moulds from the press and deposition on the cooling racks |
D | Moving the cooled moulds towards the extracting station |
E | Moving the empty moulds towards the washing station |
F | Moving the empty moulds towards the release agent application station |
Transversal requirements (valid for all the functions)
Requirement category | Constraints | Objectives |
---|---|---|
Geometry | The plant must be contained within a surface of 150 square meters | – |
Kinematics | No particular requirements. The systems required for each function should be designed independently (modularity) | – |
Material | 0.5 kg of straw needed for each mould | – |
Assembly | The assembly of the plant must be performed by experienced technicians (not a do-it-yourself plant) | – |
Transport | – | Reduce the weight of component as much as possible |
Operation | 60 wine cases per day | – |
Maintenance | – | Reduce the maintenance operation as much as possible |
Schedules | – | – |
Costs | Available budged for the pilot plant: €200k | – |
Requirements for the functions. Geometry
Function | Constraints | Objectives |
---|---|---|
Import bales | Jumbo bale: 2,100 mm × 900 mm × 1,200 mm Or Common bale: 350 mm × 500 mm × 1,200 mm Or Roll bale: D1,000 mm × 1,800 mm |
– |
Unroll bales | – | |
Cut straw fibres | Max length of fibres: 100 mm | – |
Import Mater-bi | Layer thickness 15–40 µm Width of the rolls is 800 mm Roll diameter max is 200 mm Core diameter 90 mm diam. |
– |
Roll Mater-bi + straw | Diameter of the pseudo-cylinders is 30–40 mm Length of the pseudo-cylinders 200 mm |
– |
Compress rolls into the mould | Bordolese wine bottle as a reference for dimensions The case must be realized by means of two equal parts |
– |
Heating the mould | – | Maximize the surface for the thermal conduction |
Cooling the mould | The mould must be cooled at a temperature <=40°C | – |
Extract the semi-package | – | – |
Export the semi-package | Consider a standard dimension of pallets: 800 mm × 1,200 mm |
Maximize the number of semi-packages contained into a single pallet |
Requirements for the functions. Category “Forces”
Function | Constraints | Objectives |
---|---|---|
Import bales | 330 kg max for each bale | – |
Compress rolls into the mould | 0.3 MPa max | – |
Extract the semi-package | Weight of the formed package: 1 kg Circa 1 kg per stampato |
– |
Export the semi-package | – |
Requirements for the functions. Category “signals”
Function | Constraints | Objectives |
---|---|---|
Heating the mould | It is necessary to extract the internal temperature of the moulds | – |
Cooling the mould | It is necessary to extract the internal temperature of the moulds | – |
Requirements for the functions. Category “Operation”
Function | Constraints | Objectives |
---|---|---|
Heating the mould | The heating process must be performed during the pressing of the mould | – |
References
Babalis, A., Ntintakis, I., Chaidas, D. and Makris, A. (2013), “Design and development of innovative packaging for agricultural products”, Procedia Technology, Vol. 8, pp. 575-579.
Cammarelle, A., Bimbo, F., Lombardi, M. and Viscecchia, R. (2021), “Health and eco-innovations in food packaging”, Environmental Footprints and Eco-Design of Products and Processes, Springer, Gateway, pp. 31-70.
Dahlbo, H., Poliakova, V., Mylläri, V., Sahimaa, O. and Anderson, R. (2018), “Recycling potential of post-consumer plastic packaging waste in Finland”, Waste Management, Vol. 71, pp. 52-61, doi: 10.1016/j.wasman.2017.10.033.
Davis, G. and Song, J.H. (2006), “Biodegradable packaging based on raw materials from crops and their impact on waste management”, Industrial Crops and Products, Vol. 23 No. 2, pp. 147-161.
De Pilli, T., Baiano, A., Lopriore, G., Russo, C. and Cappelletti, G.M. (2021), New Eco-Friendly Packaging Strategies Based on the Use of Agri-Food By-Products and Waste, Springer, Cham, pp. 31-41.
E.V, E.B. (2018), “BIOPLASTICS facts and figures”.
European Committee for Standardization (2010), “Plastics – Recommendation for Terminology and Characterization of Biopolymers and Bioplastics – CEN/TR 15932”, Brussels.
Ferdous, W., Manalo, A., Siddique, R., Mendis, P., Zhuge, Y., Wong, H.S., Lokuge, W., Aravinthan, T. and Schubel, P. (2021), “Recycling of landfill wastes (tyres, plastics and glass) in construction – a review on global waste generation, performance, application and future opportunities”, Resources, Conservation and Recycling, Vol. 173, p. 105745.
Ferrara, C. and De Feo, G. (2020), “Comparative life cycle assessment of alternative systems for wine packaging in Italy”, Journal of Cleaner Production, Vol. 259, p. 120888.
Fiorineschi, L., Becattini, N., Borgianni, Y. and Rotini, F. (2020a), “Testing a new structured tool for supporting requirements, formulation and decomposition”, Applied Sciences, Vol. 10 No. 9, p. 3259.
Fiorineschi, L., Papini, S., Pugi, L., Rindi, A. and Rotini, F. (2020b), “Systematic design of a new gearbox for concrete mixers”, Journal of Engineering, Design and Technology, Vol. 18 No. 6, pp. 2017-2042.
Fiorineschi, L., Frillici, F.S., Rotini, F., Conti, L. and Rossi, G. (2021a), “Adapted use of the TRIZ system operator”, Applied Sciences, Vol. 11 No. 14, pp. 1-17.
Fiorineschi, L., Bacci, T., Cubeda, S., Volpe, Y., Rotini, F., Carfagni, M. and Facchini, B. (2021b), “Non-reactive test rig for combustor-turbine interaction studies in industrial gas turbines”, Journal of Engineering, Design and Technology, Vol. 2019, doi: 10.1108/JEDT-02-2021-0085.
García-Arca, J., González-Portela Garrido, A.T. and Prado-Prado, J.C. (2017), “Sustainable packaging logistics’, the link between sustainability and competitiveness in supply chains”, Sustainability (Switzerland), Vol. 9 No. 7, p. 1098, doi: 10.3390/su9071098.
García-Arca, J., Comesaña-Benavides, J.A., Garrido, A.-P. and Prado-Prado, J.C. (2020), “Rethinking the box for sustainable logistics”, Sustainability, Vol. 12 No. 5, p. 1870, doi: 10.3390/su12051870.
Garrido, T., Etxabide, A., Leceta, I., Cabezudo, S., De La Caba, K. and Guerrero, P. (2014), “Valorization of soya by-products for sustainable packaging”, Journal of Cleaner Production, Vol. 64, pp. 228-233.
González-García, S., Gasol, C.M., Lozano, R.G., Moreira, M.T., Gabarrell, X., Rieradevall i Pons, J. and Feijoo, G. (2011a), “Assessing the global warming potential of wooden products from the furniture sector to improve their ecodesign”, Science of The Total Environment, Vols 410/411, pp. 16-25.
González-García, S., Silva, F.J., Moreira, M.T., Pascual, R.C., Lozano, R.G., Gabarrell, X., Pons, J.R.I. and Feijoo, G. (2011b), “Combined application of LCA and eco-design for the sustainable production of wood boxes for wine bottles storage”, The International Journal of Life Cycle Assessment, Vol. 16 No. 3, pp. 224-237.
Hussain, B.I. and Safiulla, M. (2018), “Comparative study of cooling systems for vacuum forming tool”, Materials Today: Proceedings, Vol. 5 No. 1, pp. 30-36.
Jansson, D.G. and Smith, S.M. (1991), “Design fixation”, Design Studies, Vol. 12 No. 1, pp. 3-11.
Ma, X. and Moultrie, J. (2018), “Understand sustainable packaging design in practice”, Proceedings of International Design Conference, DESIGN, Vol. 6, pp. 2693-2704.
Meeks, D., Hottle, T., Bilec, M.M. and Landis, A.E. (2015), “Compostable biopolymer use in the real world: stakeholder interviews to better understand the motivations and realities of use and disposal in the US”, Resources, Conservation and Recycling, Vol. 105, pp. 134-142.
Moustafa, H., Darwish, N.A. and Youssef, A.M. (2022), “Rational formulations of sustainable polyurethane/chitin/rosin composites reinforced with ZnO-doped-SiO2 nanoparticles for green packaging applications”, Food Chemistry, Vol. 371, p. 131193.
Moustafa, H., Morsy, M.A., Ateia, M.A. and Abdel-Haleem, F.M. (2021), “Ultrafast response humidity sensors based on polyvinyl chloride/graphene oxide nanocomposites for intelligent food packaging”, Sensors and Actuators A: Physical, Vol. 331, p. 112918.
Nordin, N. and Selke, S. (2010), “Social aspect of sustainable packaging”, Packaging Technology and Science, Vol. 23 No. 6, pp. 317-326.
Pahl, G., Beitz, W., Feldhusen, J. and Grote, K.H. (2007), Engineering Design 3rd ed., Springer-Verlag, London, doi: 10.1007/978-1-84628-319-2.
Pani, S.K. and Pathak, A.A. (2021), “Managing plastic packaging waste in emerging economies: the case of EPR in India”, Journal of Environmental Management, Vol. 288, p. 112405.
Petersen, K., Væggemose Nielsen, P., Bertelsen, G., Lawther, M., Olsen, M.B., Nilsson, N.H. and Mortensen, G. (1999), “Potential of biobased materials for food packaging”, Trends in Food Science and Technology, Vol. 10 No. 2, pp. 52-68, doi: 10.1016/S0924-2244(99)00019-9.
Purcell, A.T. and Gero, J.S. (1996), “Design and other types of fixation”, Design Studies, Vol. 17 No. 4, pp. 363-383.
Roozenburg, N.F. and Eekels, J. (1991), Product Design: Fundamentals and Methods, John Wiley and sons.
Rossi, G., Conti, L., Fiorineschi, L., Marvasi, M., Monti, M., Rotini, F., Togni, M. and Barbari, M. (2020), “A new eco-friendly packaging material made of straw and bioplastic”, Journal of Agricultural Engineering, Vol. 51 No. 4, pp. 185-191.
Sahu, V., Attri, R., Gupta, P. and Yadav, R. (2020), “Development of eco friendly brick using water treatment plant sludge and processed tea waste”, Journal of Engineering, Design and Technology, Vol. 18 No. 3, pp. 727-738.
Tallentire, C.W. and Steubing, B. (2020), “The environmental benefits of improving packaging waste collection in Europe”, Waste Management, The Authors, Vol. 103, pp. 426-436.
Tencati, A., Pogutz, S., Moda, B., Brambilla, M. and Cacia, C. (2016), “Prevention policies addressing packaging and packaging waste: some emerging trends”, Waste Management, Vol. 56, pp. 35-45.
Thompson-Witrick, K.A., Pitts, E.R., Nemenyi, J.L. and Budner, D. (2021), “The impact packaging type has on the flavor of wine”, Beverages, Vol. 7 No. 2, pp. 1-18.
Venkatesh, G., Ravi Kumar, Y. and Raghavendra, G. (2017), “Comparison of straight line to conformal cooling channel in injection molding”, Materials Today: Proceedings, Vol. 4 No. 2, pp. 1167-1173.
Weale, A., Pridham, G., Cini, M., Porter, D., Konstadakopulos, M. and Flynn, B. (2010), “Packaging and packaging waste”, Environmental Governance in Europe: An Ever Closer Ecological Union, Oxford university press, Oxford, doi: 10.1093/acprof.
Acknowledgements
Funding: The research has been supported by the contribution of Fondazione Cassa di Risparmio di Pistoia e Pescia to the Project “VAL.BIO.MONT.- Valorizzazione delle biomasse di scarto sul territorio montano” (Prot. n.2021.0282bl) for the funding of a 12-months research grant.