Abstract
Purpose
This paper explores the quality and flow of facade product information and the capabilities for avoiding the risk of facade fires early in the design process.
Design/methodology/approach
A qualitative case study using the process tracing method is conducted in two stages. First, a thematic analysis of reports and literature identified two categories for the problems that caused fast fire spread across the Grenfell Tower facade. This enabled classifying the identified problems into four stages of a facade life cycle: product design and manufacturing, procurement, facade design and construction. Second, the capabilities for avoiding the problems were explored by conducting in-depth interviews with 18 experts in nine countries, analyzing design processes and designers' expertise and examining the usability of three digital interfaces in providing required information for designing fire-safe facades.
Findings
The results show fundamental flaws in the quality of facade product information and usability of digital interfaces concerning fire safety. These flaws, fragmented design processes and overreliance on other specialists increase the risk of design defects that cause fast fire spread across facades.
Practical implications
The findings have implications for standardization of building product information, digitalization in industrialized construction and facade design management.
Originality/value
This research adds to the body of knowledge on sustainability in the built environment. It is the first study to highlight the fundamental problem of facade product information, which requires urgent attention in the rapid transition toward digital and industrialized construction.
Keywords
Citation
Bahrami, S. and Zeinali, D. (2023), "The sustainability challenge of product information quality in the design and construction of facades: lessons from the Grenfell Tower fire", Smart and Sustainable Built Environment, Vol. 12 No. 3, pp. 488-506. https://doi.org/10.1108/SASBE-06-2021-0100
Publisher
:Emerald Publishing Limited
Copyright © 2022, Soheila Bahrami and Davood Zeinali
License
Published by Emerald Publishing Limited. This article is published under the Creative Commons Attribution (CC BY 4.0) licence. Anyone may reproduce, distribute, translate and create derivative works of this article (for both commercial and non-commercial purposes), subject to full attribution to the original publication and authors. The full terms of this licence may be seen at http://creativecommons.org/licences/by/4.0/legalcode
Introduction
The built environment encounters sustainability problems, evident in an increasing number of facade fires worldwide (Bonner and Rein, 2020; Spearpoint et al., 2019a; White and Delichatsios, 2015). A tragic case was the Grenfell Tower fire in London in 2017. The fire claimed the lives of 72 residents and caused severe environmental contamination (Stec et al., 2019) and psychological effects on survivors, bereaved and witnesses (Cooper and Whyte, 2018). The building facade, including combustible materials, has been identified as the primary cause of the fast fire spread, heavy toxic smoke and falling debris (Lane, 2018a; McKenna et al., 2019).
Combustible facade products, commonly called sustainable building materials, have caused fires to spread fast in several green buildings (Meacham and McNamee, 2020). The attractiveness of these products relates to the changes in building codes to improve energy efficiency (McKenna et al., 2019). Although the thermal properties of these insulations can contribute to energy savings, the cost of related fires and subsequent remedies has been enormous. For example, the UK government allocated 1.6 billion pounds to replace unsafe facades [1]. This shows a lack of systems thinking (Meadows, 2008) to identify different effects of the energy-efficient strategies. Reliable product information enables designers to apply systems thinking and prevent unacceptable consequences early in the design stage of a facade life cycle.
In industrialized construction, building product manufacturers have a pivotal role in ensuring product safety by providing reliable information. This information is essential in successfully applying industrialized construction concepts, such as design for manufacturing and assembly (DfMA) and prefabrication (Alfieri et al., 2020; Lu et al., 2020). In addition, the current transition toward digitalization of construction requires digital forms of product information that are reliable (Andersson and Lessing, 2020).
Building information modeling (BIM) objects are digital forms of product information delivered by BIM object libraries to their users, including designers (Gao et al., 2017). Digital twins are other digital forms of product information. In principle, a digital twin is a dynamic virtual representation of a physical object or system across its life cycle based on real-time data (Bolton et al., 2018), enabling real-time optimization (Söderberg et al., 2017). Recent developments in digital technologies have triggered several studies on digital twins of building elements (e.g. Khajavi et al., 2019; Lydon et al., 2019).
Prior research has shown the problem of deficient information concerning the sustainability performance of building ventilation products (Bahrami et al., 2019b). The primary cause is the unstandardized methods manufacturers use for presenting product information, which has also affected the BIM objects (Bahrami et al., 2019a; Pasini et al., 2017). Regarding facade products, several scholars have studied the effect of facade materials on fire spread (e.g. Hidalgo et al., 2017; McKenna et al., 2019; Nguyen et al., 2020). However, no studies to date have investigated the quality and flow of information on the fire safety of facade products. Also, previous studies have not examined the usability of digital interfaces concerning the fire safety of facades.
This study aims to investigate the quality and flow of facade product information concerning fire safety. A qualitative case study approach has been adopted to explore the design defects underlying fire spread in facades and the capabilities for avoiding those defects.
In the following sections, the paper first reviews the literature on the Grenfell Tower fire, the problem of facade fires and building product information and sustainable design. Next, it describes the methodology applied in this study. The subsequent section discusses the findings in three subsections. The first subsection categorizes the design failures in the Grenfell Tower re-cladding project and argues the credibility of related research publications. The second subsection identifies the problems associated with product information in four stages of a facade product life cycle: product design and manufacturing, procurement, facade design and construction. In the third subsection, the capabilities for avoiding the identified problems are examined. Finally, the paper draws conclusions from the findings and provides recommendations for necessary improvements and further research.
Literature review
The Grenfell Tower fire
The Grenfell Tower, a 24-story residential building, was renovated from 2012 to 2016 (Potton and Sutherland, 2020). To improve energy efficiency in the building, an insulated facade was installed on its exterior wall (McKenna et al., 2019). However, on 14 June 2017, a fire started in an apartment on the fourth floor of the building, broke into the facade, propagated through the facade assembly rapidly and entered the other apartments (Bisby, 2018; Torero, 2018). In just 18 min, the fire spread up through 20 stories to the roof of the building (Lane, 2018a). Research and investigations show that the defects in building facade design and construction were the leading causes of a fast fire spread that produced heavy toxic smoke and falling debris (Lane, 2018a; McKenna et al., 2019).
The facade was a ventilated rain screen cladding assembly comprising aluminum composite panels (ACP) with combustible polyethylene (PE) core, cladding rails, joints, cavity barriers, and combustible insulation boards (Lane, 2018a). The polyisocyanurate (PIR) foam and phenolic foam insulation boards, along with the missing and defective cavity barriers, became part of a sustained combustion process generating fire spread over six distinguished pathways through the facade (Lane, 2018a). The fire entered the apartments through newly installed window frames made of aluminum with combustible Styrofoam (extruded polystyrene) insulating core, combustible unplasticized polyvinyl chloride (UPVC) linings and weatherproof membranes made of combustible ethylene propylene diene monomer (EPDM) (Lane, 2018b, e).
During on-site investigations after the fire incident, Lane (2018c) identified PIR insulation boards of 25 mm thickness fixed to the back of 10 mm thick UPVC linings, although they were not shown in the design drawings. Burning PIR generates dangerous amounts of hydrogen cyanide (HCN) and carbon monoxide (CO) (McKenna et al., 2019). According to the investigation reports on the Grenfell Tower fire, smoke delayed or prevented residents from entering the escape stair (Lane, 2018a).
The problem of facade fires
Unfortunately, the Grenfell Tower tragedy was not the first fire incident associated with facade issues, nor was it the last one. The past three decades have witnessed a growing number of facade fires worldwide (see Figure 1).
This is a continuing concern because of the combustible facade products installed on many existing and new buildings. An example is The Cube fire in Bolton, the UK, in 2019. According to the Greater Manchester Fire and Rescue Service (2019), the fire spread rapidly through the high-pressure laminate (HPL) facade, seriously endangered 217 residents' lives, and caused severe property damage and significant disruptions in the students' studies. Moreover, all on-duty supervisory officers and 90% of available fire engines were occupied at the peak of the operational activity (Greater Manchester Fire and Rescue Service, 2019). Avoiding the risk of such dangerous fires requires reliable flows of information on the fire resistance of facade products.
Building product information and sustainable design
Since the 1990s, designing products with low environmental impacts has received attention (Vezzoli et al., 2018) and inspired design approaches such as green design, eco-design and product life cycle design (Ceschin and Gaziulusoy, 2016; Vezzoli et al., 2018). However, limited attention has been given to the human-related aspects (Ceschin and Gaziulusoy, 2020). This flaw is evident in sustainable designs promoting insulation materials that can increase energy efficiency but cause fatal facade fires. Facade designers need systems thinking to identify the adverse impact of the strategies that have been considered green or sustainable. Systems thinking enables us to understand the elements, see their interactions, ask what-if questions to predict their future behaviors and redesign the existing systems (Meadows, 2008). It provides a holistic design framework for creating change toward sustainable solutions (Wright and Ceroni, 2017, p. 15).
The flow of information plays a central role in determining how a system operates, and reliable information ensures the integrity of a system (Meadows, 2008). Therefore, sustainable facade designs need an operative flow of reliable information on the sustainability performance of products. This is of particular importance in facade design and manufacturing, which requires the early integration of several design criteria characterized by cross-disciplinary interdependencies and manufacturing constraints (Montali, 2019, p. 14). For example, a facade contractor and 11 subcontractors delivered the Grenfell Tower re-cladding project (Lane, 2018d). In addition, designers' knowledge and experience are significant in ensuring the adequacy and correctness of product information. O'Connor and Koo (2021) suggested that designers might not detect missing information because of insufficient knowledge and experience.
Despite its significant role, the provision of building product information has been identified as inadequate and inefficient (Pasini et al., 2017), particularly concerning the sustainability attributes of products (Krueger et al., 2019) and BIM objects of ventilation products (Bahrami et al., 2019a). Also, unstandardized methods adopted by manufacturers for presenting product information have limited the usability of BIM object libraries (Pasini et al., 2017). Previous studies have not investigated the provision of facade product information and the quality of their BIM objects.
Standardization of product information enhances the exchange of information, communication (Ho and O'Sullivan, 2015) and transparency (Egyedi and Ortt, 2017) among various stakeholders and increases market penetration (Tassey, 2017). In particular, providing standardized information on the sustainability attributes of products allows for decisions that are more informed and sustainable (Cho et al., 2018). To support sustainability in the built environment, the standards on product information must promote the identification of the sustainability attributes and comparison of products (Bahrami et al., 2019b).
Sustainable designs require information on the entire product life cycle. The concept of digital twins has been developed to provide reliable information throughout the product life cycle (Grieves and Vickers, 2017). The idea of utilizing digital twins in manufacturing is to determine the manufacturability and failure modes before producing a physical system to reduce costs, time and harm to users (Grieves and Vickers, 2017). The concept of digital twins has also attracted attention in research on the operation phase of facades (e.g. Böke et al., 2020; Khajavi et al., 2019). However, no study has explored the application of digital twins in facade design.
The quality and flow of building product information are significant in industrialized construction (Dubois et al., 2019), characterized by product orientation (Andersson and Lessing, 2020; Kedir and Hall, 2021). Industrialized construction can facilitate information management through standardized processes (Eriksson et al., 2019) and mainly relies on DfMA and prefabrication (Alfieri et al., 2020; Lu et al., 2020), which have been studied in the design and construction of facades (Chen and Lu, 2018; Montali et al., 2018).
Applying DfMA in facade system projects (e.g. Chen and Lu, 2018) has reduced planning and construction work and improved safety, quality and reliability (Chen and Lu, 2018). Currently, prefabrication of facades involves installing fire stops and cavity barriers on-site, which increases the risk of fire spread caused by incorrect installations (Nguyen et al., 2020). In addition, during the installation of prefabricated facades, limited accuracy of lifting cranes might cause misalignments that incite fire spread across the building (Nguyen et al., 2020). Therefore, adopting a DfMA approach can effectively address the assembly problems in the design stage of a building product life cycle (Gao et al., 2020; Lu et al., 2020).
The quality of product information affects the designer's choice of products, and thus, plays a pivotal role in the effectiveness of DfMA and prefabrication. However, the fundamental questions regarding the quality of facade product information delivered by manufacturers have remained unanswered. In addition, successful industrialization and digitalization of construction require a reliable supply of digital product information (Andersson and Lessing, 2020). This highlights the need for a thorough understanding of the usability of digital interfaces that deliver product information.
The quality of information depends on its accuracy, precision, currency, sufficiency, comprehensibility, timeliness, relevance and usability (Peter et al., 2013), while the essential quality of information is its truthfulness (Floridi, 2019). The interface usability can be evaluated by the searchability (Whyte, 2019), traceability (Eppler, 2006, p. 71), availability and comparability of the information delivered by the interface (Bahrami et al., 2019a).
Methodology
This study has employed a qualitative case study approach. Qualitative research is a fruitful way of providing insight into the existing or emerging concepts by using various sources of information (Yin, 2016). Conducting a case study is a rational approach in qualitative research (Creswell and Poth, 2018).
To design the case study, a comprehensive review and thematic analysis of the documents and literature on the facade fires have been conducted to understand the most common factors involved in those incidents. This process is complemented by analyzing a database on facade fires created by Spearpoint et al. (2019b). The results show that the material and type of facades were the underlying causes of fast fire spread and the production of heavy smoke and falling debris, which restrained firefighting operations, prevented escape and led to tragic consequences.
Based on these findings, a case study with embedded units of analysis (Yin, 2018) has been designed. The first and fundamental unit of analysis is product information regarding fire safety performance. Other units are three elements in the flow of product information: digital interfaces providing facade product information, design processes and designers' expertise. The inspiration for selecting these three elements is gained from Toyota's eighth management principle that emphasizes technology to support processes and people in a product development system (Liker, 2020, p. 7).
The case study has been conducted in two main stages. The first stage aims to identify the potential design defects that can cause dangerous facade fires. The Grenfell Tower fire is selected as a prominent example. In addition, the results of two comprehensive studies on design defects (O'Connor and Koo, 2020, 2021) have been used to examine the applicability of the findings from the Grenfell Tower case in the general context of design and construction. Then, an analysis strategy is developed by integrating the data analysis spiral (Creswell and Poth, 2018) into the explaining outcome process tracing method (Beach and Pedersen, 2016). The analysis steps are as follows.
Organizing and coding the data
Categorizing the codes into four themes (product information, design procurement and implementation processes, designers' expertise and digital interfaces),
Relating the themes to the outcome (dangerous facade fires)
Visualizing the data
Proposing sufficient explanations for the causal mechanisms
Writing an account of findings
This strategy is adopted to analyze the data collected from the following sources.
Nine detailed investigation reports by four expert witnesses (947 pages in total)
Product test documents (122 pages)
Approximately 17 h of inquiry hearing videos and transcripts
21 research articles and two dissertations regarding the design aspects of the Grenfell Tower facade
Two comprehensive studies on design defects
The second stage of the case study explores the capabilities for avoiding the failures identified in the first stage. This stage encompasses qualitative review and analysis of the data collected from the sources listed in Table 1.
As indicated in Table 1, the second stage of the study firstly includes semi-structured in-depth interviews conducted with 18 experts. Their professions are facade engineer, fire and risk engineer, product development manager, manufacturing research engineer, industrialized construction director, automation director, IoT director, researcher (digital twins) and digitalization manager. Their organizations are located in Australia, Denmark, Finland, France, Italy, Sweden, the UAE, the UK; and the USA, the UAE, the UK and Australia are selected because of the considerable impact of facade fires on the construction industry in those countries. The experts in Italy have been involved in facade projects in the UAE and UK. Those located in other countries are selected based on their expertise in manufacturing and the digital technologies covered by this study.
The second stage followed the six steps of data analysis used in the first stage. Regarding the fourth unit of analysis (digital interfaces), three digital tools are selected to examine their usability in providing information for avoiding design defects. These tools are the NFPA's interactive tool, a set of three BIM object libraries and Ansys GRANTA EduPack (see Table 1). The NFPA's tool is selected because it is a tool that can help users explore the code requirements and learn about the information that facade product manufacturers must provide on the fire safety performance of their products. For testing the usability of the tool, two experts followed the design scenario below in 50 trials (all possible paths).
Building code: International Building Code (IBC)
Facade type: Non-load-bearing exterior wall
Construction type: Type I (building structure is made of non-combustible or limited-combustible materials, mainly concrete and steel)
Facade material: containing a combustible MCM (e.g. ACP with PE core)
Installation height: More than 22.86 meters
The BIM object libraries (BIMObject, BIMStore and NBS National BIM Library) are selected based on their popularity and the number of facade BIM objects they provide. In total, 50 BIM objects of facade components, including ACPs and insulation foams available in the libraries, are analyzed.
Material databases play a significant role in providing both product designers and building designers with information on fire risks of materials. The MaterialUniverse database is a reliable resource for materials scientists and engineers (NASA, 2021). This database can be accessed through the Ansys GRANTA software, widely used in product development and materials research and education. Therefore, GRANTA EduPack, the educational version of Ansys GRANTA, is selected to study. To examine the capability of the software for providing information on the fire safety of products, a material selection scenario comprising the following steps has been designed. A total of two experienced users followed these steps in two trials. Next, a highly experienced user repeated the trial to confirm the findings.
Selection of the architecture data set
Search for both the PE core and the ACP assembly
Search for the available information regarding fire safety on the generic datasheets and the manufacturers' datasheets
Search for non-flammable alternatives: narrowing down the search by the thermal resistivity range of PE as an indicator of energy efficiency
Iterating steps 2 to 4 using the advanced (level 3) data set
In order to analyze the usability of the selected digital tools and the quality of the information they provide, a set of criteria is determined by modifying the information quality frameworks suggested by Eppler (2006, p. 68) and Bahrami et al. (2019a) in line with the purpose of this study (see Figure 2).
For all three digital tools selected to study, the information quality is analyzed using the criteria in Figure 2. However, the purpose of the NFPA's interactive tool is “to help navigate the code requirements that apply to exterior walls containing combustible components” [2], not to provide information on specific products. Therefore, to examine the NFPA's tool, the criteria presented in Figure 2 for evaluating interface quality are replaced with “navigation functionality”. This criterion is analyzed by creating a flowchart process map, presented and discussed in the next section. Also, “consistency” is not considered for evaluating the tool because it does not provide information on manufacturers' products.
Findings and discussion
The analysis of the reported facade fires shows that the facade type and the combustibility of the materials (e.g. insulation and ACP core) were the most common factors involved in those incidents. For example, 42% of the known facade types were metal composite panels, and 40% of them were exterior insulation and finish systems. All the ACPs in the reported fire incidents had PE core, and 90% of the known insulation materials were combustible. The facade type in 27% of the reported incidents and the insulation materials in 63% of the reported incidents were unknown.
Fundamental design failures in the Grenfell Tower re-cladding
Table 2 lists the significant issues regarding product information, design and procurement processes, designers' expertise and digital tools in the Grenfell Tower facade project.
In several research publications, an oversimplification of the structure of the Grenfell Tower facade shows an air gap of 50 mm, and that size is identified as the dominant factor in the fast fire spread. However, investigation reports show that cavities such as a 20 mm gap at the head of the windows mentioned in (Lane, 2018b, Clause 8.10.7) and the defective installation of cavity barriers mentioned in the investigation report (Lane, 2018e, Clause 10.3.44) created a connected network of cavities with different sizes in the facade system.
The analysis of the related research publications revealed a series of studies with incomplete conclusions based on simplified models of the facade system (e.g. Guillaume et al., 2020). For instance, the combustibility of the phenolic foam has been neglected, while McKenna et al. (2019) reported that compared to the least combustible insulation materials, burning phenolic foam showed 48 times greater total heat release and produced five times more toxic smoke. Another example is modeling the Grenfell Tower's window system without EPDM waterproof membranes by Koohkan et al. (2020), which in the investigator's report (Lane, 2018e, Clause 10.3.26) was identified as one of the main materials causing the vertical fire spread in the Grenfell Tower facade. These findings show that scientific papers need more rigorous and critical evaluation when consulted in design or regulatory decisions.
The potential problems during the design and construction of a facade
The identified failures (Table 2) and the defects identified by O'Connor and Koo (2020) have been used to determine the problems that can occur during the flow of product information throughout the design and construction stages of a facade life cycle (see Figure 3).
What stands out in Figure 3 is that misleading product information can flow throughout a facade life cycle because of limited knowledge or professional negligence of designers, fire engineers and inspectors. This issue might not be detected due to the lack of adequate documentation control and coordination among various subcontractors involved in the design process.
Exploring the capabilities for avoiding the identified problems
The second stage of this study has investigated the causes of the problems identified in the first stage and the capabilities for avoiding those problems.
On the theme of product information, the interviewees are concerned about the availability, understandability and validity of the information received from manufacturers. Accurate information on the properties and installation of facade components enables designers to evaluate the constructability and fire safety of their designs. According to the interviewees experienced in industrialized construction, DfMA provides this information effectively because it addresses the installation (assembly) issues early in the design process. They also argued that DfMA creates consistency in the supply chain because the requirements for products can be consistently articulated, requested and controlled.
Concerning the design process, a facade engineer mentioned, “a lot of opportunities are missed for the effective exchange of information and design optimization due to the fact that the design process is very segmented (at least in the UK). It is not uncommon that a company advises for early design development (RIBA stage 1–2), then a different company works for stage 3, and then a different company does the technical design (stage 4).”
Regarding the designers' expertise, the analysis of the facade professionals' profiles shows that graduates in various areas, including facade engineering, architecture, structural engineering, architectural engineering and building physics, work as facade designers or facade engineers depending on the services offered by their employers. Therefore, there is an essential need for work experience and professional certifications to ensure that facade designers have gained an adequate understanding of fire safety. In the UAE, the required qualifications for facade specialists include five years' experience in fire safety of facades and certification by Civil Defense (General Headquarters of Civil Defense UAE, 2018).
The findings also show the effect of the professional experience on the fire safety of designs. The senior designers expressed their concerns regarding the adequacy, accuracy and understandability of product information, particularly fire test reports. However, a junior designer mentioned that product manufacturers provide reliable information on their products; thus, it is unnecessary to review the details in the product documents. One of the senior facade engineers believes that reviewing the drawings provided by architects followed by on-site inspections is necessary to avoid fire risk and liability problems. However, junior designers assume that the architects or other specialists would eventually control the work for errors. This is what O'Connor and Koo (2021) mentioned as a “tendency to transfer responsibility to others” and “overreliance on others”. The Grenfell Tower facade designer had a similar approach regarding the compliance of the design decisions with the fire safety codes (Lamb, 2018, statement 56). Although preparing a responsibility matrix has been recognized as a solution (Hyett, 2020a; Royal Institute of British Architects, 2020), the interviewees suggest that its successful implementation depends on the individual's knowledge and understanding of the task.
On the theme of digital interfaces, the NFPA's tool, three BIM object libraries and GRANTA EduPack are used to investigate the usability of these interfaces in the fire-safe design of facades. The NFPA's tool provides information on the NFPA 5000 and IBC requirements applicable to different design choices. This information can improve the knowledge of code requirements among facade designers and those involved in the design and manufacturing of facade products. Through exploring the paths, users can compile a list of information that manufacturers must provide, for example, standard tests for different performance criteria (e.g. UL 723 for flame spread index for an MCM facade) and solutions for fire containment and extinguishment (e.g. flame barriers). The flowchart map in Figure 4 illustrates the results of 50 trials following the scenario described previously in the methodology section.
Three major defects have been detected in the navigation functionality. First, as shown in Figure 4, at point B, the user is asked whether the building is equipped with an automatic sprinkler system; if the answer is “Yes”, there is a path to option 2. However, at point C, the same question is repeated with an option if the answer is “No”. Second, it is not possible to go backward and change the design options in the same path (e.g. in the bold path in Figure 4, instead of going back from point E to point D, the user must start again from point A). Third, there are nonfunctioning buttons (e.g. the error sign in Figure 4). Concerning the information quality, the tool content clearly explains the product information that facade products manufacturers must provide (bold text in the flowchart boxes in Figure 4). Explanatory notes clarify the terminology, and the information has been accurately retrieved from the code sections mentioned on each page. The information is adequate for gaining a broad knowledge of the code requirements applied to various facade design options. However, the information is outdated (based on the 2015 editions of IBC and NFPA 5000). The results of the analysis are summarized in Table 3. The results indicate that the usability of the tool is limited by outdated information and functional defects in the navigation structure.
The evaluation of the BIM object libraries is conducted by analyzing 50 manufacturers' BIM objects of facade components, including ACPs and insulation foams in three BIM object libraries. The results show that the information on the fire safety of products must be accessed through links to the manufacturers' websites, while the links are not provided in 64% of the BIM objects. Moreover, it is not possible to search for facade products and compare them based on the criteria for fire safety performance. Another limitation is that the changes in the product specifications relating to fire safety cannot be traced.
In addition, this study has identified fundamental problems relating to the quality of the information in the BIM object contents. First, the information is not understandable because of undescribed terminologies and notations. In 5% of the objects for the global market, the information is not provided in English. Second, despite the availability of information about the date of the edition and the revision date, this information is not an indicator of whether the product information is up-to-date. For example, for a BIM object updated in 2020, the information provided by a manufacturer referred to a withdrawn standard (BS 476: Part 7: 1987). Third, the accuracy of the information on fire safety performance cannot be confirmed in 96% of the analyzed BIM objects because manufacturers have not provided valid certificates or fire test results for those products. As a result, none of the analyzed BIM objects includes adequate information for selecting fire-safe products. Finally, the methods of representing the information on the fire safety of products are inconsistent across different manufacturers. These findings, summarized in Table 3, indicate that the poor quality of the manufacturers' product information has limited the usability of the BIM object libraries in delivering information on the fire safety of facade products.
The transition toward industrialized construction and DfMA requires reliable sources of information on the construction materials and usable interfaces that can be used in both manufacturing and building design. The Ansys GRANTA EduPack is used in this study to access the MaterialUniverse database, which contains information on approximately 4000 types of materials in the global market. The database is known as an outstanding resource for scientists and engineers in the field of materials (NASA, 2021). During three trials, this study could not find the desired information in the architecture dataset in GRANTA. Therefore, the advanced dataset has also been used in each of the three trials described in the methodology section.
The results show that information on the fire safety of facade products is extremely limited. For ACPs, there is just one record with a link to just three manufacturers' information pages. The interface enables searching for non-flammable materials, but the flammability classification is provided in the general information for a type of material, not for a specific manufacturer's product. The reason is the limited information provided by manufacturers, which has also inhibited the comparability of products. Therefore, the information does not suffice for comparing products based on their fire safety performance, although the interface has valuable functions for comparing materials. Similar to BIM library objects, the interface provides links to the manufacturers' websites but does not enable users to trace the changes in the product specifications relating to fire safety.
Explanatory notes are provided for the terminology. For example, “flammability” is explained in a design note, and related theoretical knowledge is briefly described in a science note. The database is updated annually, but the product information cannot be updated if the manufacturer does not provide current information. A notable problem is the accuracy of the information regarding fire safety. For instance, in the architecture data set, ACP is classified as “self-extinguishing”, referred to ASTM E1264, the standard for classifying acoustical ceiling products, not facade products. In the advanced dataset, it is classified as “slow-burning” based on UL 94 (a standard for flammability ratings).
The information provided by the software is collected from standards, handbooks, journal publications, third-party data modules and manufacturers' datasheets. On the information pages, it is mentioned that the software provider gives no warranty for the data. Regarding the adequacy of the information, the results indicate that the available information is not adequate for selecting fire-safe products. In addition, the inconsistent methods used by manufacturers for representing the information on the fire safety of their products similarly affected the MaterialUniverse database and the BIM object libraries. Table 3 shows the results of the evaluation of the MaterialUniverse database provided by GRANTA EduPack regarding its usability in the fire-safe design of facades. The findings imply that although the software has great potential to support material selection for sustainable design, the poor quality of manufacturers' product information has impeded its usability for the design and manufacturing of fire-safe facade products.
Furthermore, the available publications on the application of digital twins for facades have been investigated through four interviews with experts who either authored the publications or were involved in the reported projects on digital twins of facades. The findings show that digital twins are still not applied in the design of facades due to technological limitations. However, recent advances in platforms such as Microsoft Azure Digital Twin and Autodesk Tandem can catalyze the creation of facade digital twins, thereby improving the creation and flow of product information.
Conclusions and recommendations
This study has aimed to explore the quality and flow of facade product information and the capabilities for avoiding the risk of facade fires early in the design stage of a facade life cycle. The findings have implications for the standardization of building product information, facade design management and digitalization in industrialized construction. In addition, this work contributes to the research on sustainability in the built environment by developing a framework for evaluating the product information quality in digital tools and databases.
The findings show that deficient information provided by manufacturers has hindered digital tools from delivering the information required for designing fire-safe facades. This can be explained by the lack of standards and regulations on providing information regarding the fire safety of facade products. In addition, the standards and regulations must address the information concerning installation instructions.
The variety of suppliers and the fragmentation of design processes can impede the effective flow of product information, cause design defects and increase the risk of facade fires. The DfMA approach and product orientation in industrialized construction have been suggested as possible solutions for improving coordination in design and creating product-safety-oriented ecosystems in the construction industry. However, this requires integrating the sustainability objectives, particularly the fire safety of facades, into DfMA and providing manufacturers and facade designers with the required knowledge.
This study suggests that to ensure the fire safety of facades, all designers involved in design processes, including product design and manufacturing, architectural, structural, mechanical and electrical design, must have a proper understanding of materials and fire safety of buildings. Systems thinking is necessary to understand the facade components as parts of a system and consider their interactions with each other and their surroundings over time. In addition, it is essential to understand the interactions between various design strategies for sustainability, such as energy-efficient solutions and fire safety requirements. Moreover, a basic understanding of the fire test standards enables facade designers to detect information problems in product datasheets and avoid overreliance on other specialists in the design process. Professional certification programs would also help reduce the risk of inadequate knowledge of fire safety.
Developing effective test methods and criteria for evaluating the fire safety of facade products requires reliable research results. However, incomplete conclusions in the research publications that were analyzed in this study might mislead legislation, standardization and design processes. Therefore, more collaborative studies on the fire safety of facades are needed to achieve unbiased results that can be consulted in these processes.
In this study, investigating the digital tools is limited to the NFPA's exterior walls interactive tool, BIM objects and GRANTA EduPack. Further research is recommended to investigate other digital tools and databases that facade designers use commonly. Finally, the findings of this study indicate that digital twins are currently not used in facade design, even though their application aims to ensure product safety in the design stage. Future research on this topic is therefore recommended.
Figures
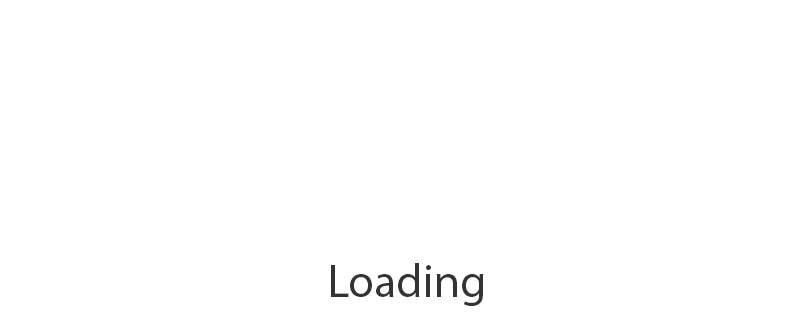
Figure 1
Reported facade fire incidents (the data has been collected from Bonner and Rein, 2020, Spearpoint et al., 2019b; White and Delichatsios, 2015)
The sources of data collection in the second stage of this study
Semi-structured interviews with 18 experts Professional profiles of 50 facade experts Exterior walls interactive tool, developed by the national fire protection association (NFPA)1 A collection of 50 BIM objects of facade systems and components provided on 3 BIM object libraries (BIMObject2, BIMStore3 and NBS national BIM library4) Ansys GRANTA EduPack5 |
RIBA plan of work 2020 (Royal Institute of British Architects, 2020) |
Fire performance of external walls and cladding (Australian Building Codes Board, 2020) |
Guide for the assessment of buildings with combustible cladding (New South Wales Department of Planning Industry and Environment, 2019) |
The UAE fire and life safety code of practice (General Headquarters of Civil Defense UAE, 2018) |
A categorization of the defects in the Grenfell Tower facade project
Product information |
|
Design and procurement processes, designers' expertise and digital interfaces |
|
The provision of information on the fire safety performance of facade products
NFPA tool | BIM object libraries | MaterialUniverse | ||
---|---|---|---|---|
Interface usability | Navigation functionality | Defective | N/A | N/A |
Availability | N/A | Very limited | Extremely limited | |
Searchability | N/A | Unattainable | Unattainable | |
Comparability | N/A | Unattainable | Unattainable | |
Traceability | N/A | Unattainable | Unattainable | |
Information quality | Understandability | Clarified terms | Unclear | Clarified terms |
Currency | Outdated | Uncertain | Uncertain | |
Accuracy | Accurate | Inaccurate | Inaccurate | |
Adequacy | Adequate | Inadequate | Inadequate | |
Consistency | N/A | Inconsistent | Inconsistent |
Note(s): N/A: Not applicable to this tool
Notes
References
Alfieri, E., Seghezzi, E., Sauchelli, M., Di Giuda, G.M. and Masera, G. (2020), “A BIM-based approach for DfMA in building construction: framework and first results on an Italian case study”, Architectural Engineering and Design Management, Vol. 16 No. 4, pp. 247-269, doi: 10.1080/17452007.2020.1726725.
Andersson, N. and Lessing, J. (2020), “Industrialization of construction: implications on standards, business models and project orientation”, Organization, Technology and Management in Construction, Vol. 12 No. 1, pp. 2109-2116, doi: 10.2478/otmcj-2020-0007.
Anketell-Jones, D. (2019), “Grenfell tower public inquiry-witness statement”, available at: https://assets.grenfelltowerinquiry.org.uk/HAR00010513_2019.03.19 Daniel Anketell-Jones supplementary statement %28Harley Facades%29.pdf (accessed 28 November 2021).
Australian Building Codes Board (2020), “Fire performance of external walls and cladding”, available at: https://www.abcb.gov.au/Resources/Publications/Education-Training/Fire-performance-of-external-walls-and-cladding (accessed 28 November 2021).
Bahrami, S., Atkin, B. and Landin, A. (2019a), “Enabling the diffusion of sustainable product innovations in BIM library platforms”, Journal of Innovation Management, Vol. 7, pp. 106-130, doi: 10.24840/2183-0606_007.004_0006.
Bahrami, S., Atkin, B. and Landin, A. (2019b), “Innovation diffusion through standardization: a study of building ventilation products”, Journal of Engineering and Technology Management, Vol. 54 No. 4, pp. 56-66, doi: 10.1016/j.jengtecman.2019.11.001.
Beach, D. and Pedersen, R.B. (2016), Causal Case Study Methods: Foundations and Guidelines for Comparing, Matching and Tracing, University of Michigan Press, Ann Arbor. doi: 10.3998/mpub.6576809.
Bisby, L. (2018), “Grenfell tower inquiry: phase 1 - expert report”, available at: https://assets.grenfelltowerinquiry.org.uk/documents/Professor%20Luke%20Bisby%20report%20%28Phase%201%20-%20supplemental%29%20-%20LBYS0000001.pdf (accessed 28 November 2021).
Böke, J., Knaack, U. and Hemmerling, M. (2020), “Prototype of a cyber-physical façade system”, Journal of Building Engineering, Vol. 31, 101397, doi: 10.1016/j.jobe.2020.101397.
Bolton, R.N., McColl-Kennedy, J.R., Cheung, L., Gallan, A., Orsinghe, C., Witell, L. and Zaki, M. (2018), “Customer experience challenges: bringing together digital, physical and social”, Journal of Service Management, Vol. 29 No. 5, pp. 776-808, doi: 10.1108/JOSM-04-2018-0113.
Bonner, M. and Rein, G. (2020), “List of facade fires 1990-2020”, available at: https://zenodo.org/record/3743863 (accessed 28 November 2021).
Ceschin, F. and Gaziulusoy, I. (2016), “Evolution of design for sustainability: from product design to design for system innovations and transitions”, Design Studies, Vol. 47, pp. 118-163, doi: 10.1016/j.destud.2016.09.002.
Ceschin, F. and Gaziulusoy, İ. (2020), Design for Sustainability: A Multi-Level Framework from Products to Socio-Technical Systems, Routledge, New York, NY. doi: 10.4324/9780429456510.
Chen, K. and Lu, W. (2018), “Design for manufacture and assembly oriented design approach to a curtain wall system: a case study of a commercial building in Wuhan, China”, Sustainability, Vol. 10 No. 7, p. 2211, doi: 10.3390/su10072211.
Cho, Y.N., Soster, R.L. and Burton, S. (2018), “Enhancing environmentally conscious consumption through standardized sustainability information”, Journal of Consumer Affairs, Vol. 52 No. 2, pp. 393-414, doi: 10.1111/joca.12172.
Cooper, V. and Whyte, D. (2018), “Grenfell, austerity, and institutional violence”, Sociological Research Online, pp. 1-10, doi: 10.1177/1360780418800066.
Creswell, J.W. and Poth, C.N. (2018), Qualitative Inquiry and Research Design: Choosing Among Five Approaches, 4th ed., Sage Publications, Los Angeles, 9781506361178.
Dubois, A., Hulthén, K. and Sundquist, V. (2019), “Organising logistics and transport activities in construction”, International Journal of Logistics Management, Vol. 30 No. 2, pp. 620-640, doi: 10.1108/IJLM-12-2017-0325.
Egyedi, T.M. and Ortt, J.R. (2017), “Towards a functional classification of standards for innovation research”, in Hawkins, R., Blind, K. and Page, R. (Eds), Handbook of Innovation and Standards, Edward Elgar, Cheltenham, pp. 105-131, ISBN 978-1-78347-008-2.
Eppler, M.J. (2006), Managing Information Quality, Springer, Berlin. doi: 10.1007/3-540-32225-6.
Eriksson, H., Sandberg, M., Mukkavaara, J., Jansson, G. and Stehn, L. (2019), “Assessing digital information management between design and production in industrialised house-building – a case study”, 2019 Proceedings of the 36th ISARC, Banff, Canada, pp. 340-347, doi: 10.22260/isarc2019/0046.
Floridi, L. (2019), “Information quality”, The Logic of Information: A Theory of Philosophy as Conceptual Design, Oxford University Press, Oxford, pp. 101-112. doi: 10.1093/oso/9780198833635.003.0005.
Gao, G., Liu, Y.S., Lin, P., Wang, M., Gu, M. and Yong, J.H. (2017), “BIMTag: concept-based automatic semantic annotation of online BIM product resources”, Advanced Engineering Informatics, Vol. 31, pp. 48-61, doi: 10.1016/j.aei.2015.10.003.
Gao, S., Jin, R. and Lu, W. (2020), “Design for manufacture and assembly in construction: a review”, Building Research and Information, Vol. 48 No. 5, pp. 538-550, doi: 10.1080/09613218.2019.1660608.
General Headquarters of Civil Defence UAE (2018), “UAE fire and life safety code of Practice”, available at: https://www.dcd.gov.ae/portal/eng/UAEFIRECODE_ENG_SEPTEMBER_2018.pdf (accessed 28 November 2021).
Greater Manchester Fire and Rescue Service (2019), “The Cube: incident report and key observations regarding the fire which occurred on 15 november 2019”, available at: https://www.manchesterfire.gov.uk/media/2118/cube_report_v11_tagged.pdf (accessed 28 November 2021).
Grieves, M. and Vickers, J. (2017), “Digital twin: mitigating unpredictable, undesirable emergent behavior in complex systems”, in Kahlen, F.J., Flumerfelt, S. and Alves, A. (Eds), Transdisciplinary Perspectives on Complex Systems: New Findings and Approaches, Springer, pp. 85-113, doi: 10.1007/978-3-319-38756-7_4.
Guillaume, E., Dréan, V., Girardin, B., Benameur, F. and Fateh, T. (2020), “Reconstruction of Grenfell Tower fire. Part 1: lessons from observations and determination of work hypotheses”, Fire and Materials, Vol. 44 No. 1, pp. 3-14, doi: 10.1002/fam.2766.
Hidalgo, J.P., Torero, J.L. and Welch, S. (2017), “Experimental characterisation of the fire behaviour of thermal insulation materials for a performance-based design methodology”, Fire Technology, Vol. 53, pp. 1201-1232, doi: 10.1007/s10694-016-0625-z1.
Ho, J.Y. and O'Sullivan, E. (2015), “The evolving role of standardisation in technological innovation: the case of photovoltaics”, 2015 IEEE 9th International Conference on Standardization and Innovation in Information Technology (SIIT), IEEE, pp. 1-8, doi: 10.1109/SIIT.2015.7535597.
Hyett, P. (2020a), “Grenfell tower inquiry- Paul Hyett, H KS Architects Ltd supplemental report Part 1”, available at: https://assets.grenfelltowerinquiry.org.uk/PHYS0000002_Part 1 of Paul Hyett%27s Supplemental Report.pdf (accessed 28 November 2021).
Hyett, P. (2020b), “Specialist report-section 6-failures in quality control”, available at: https://assets.grenfelltowerinquiry.org.uk/PHYR0000031_Section 6_ Failures in Quality Control %28Paul Hyett%27s Amended Report%29.pdf (accessed 28 November 2021).
Kedir, F. and Hall, D.M. (2021), “Resource efficiency in industrialized housing construction – a systematic review of current performance and future opportunities”, Journal of Cleaner Production, Vol. 286, 125443, doi: 10.1016/j.jclepro.2020.125443.
Khajavi, S.H., Motlagh, N.H., Jaribion, A., Werner, L.C. and Holmstrom, J. (2019), “Digital Twin: vision, benefits, boundaries, and creation for buildings”, IEEE Access, Vol. 7, pp. 147406-147419, doi: 10.1109/ACCESS.2019.2946515.
Koohkan, M., Dréan, V., Girardin, B., Guillaume, E., Fateh, T. and Duponchel, X. (2020), “Reconstruction of the Grenfell tower fire – thermomechanical analysis of window failure during the Grenfell tower disaster”, Fire Technology, Vol. 57, pp. 69-100, doi: 10.1007/s10694-020-00980-4.
Krueger, K., Stoker, A. and Gaustad, G. (2019), “Alternative materials in the green building and construction sector: examples, barriers, and environmental analysis”, Smart and Sustainable Built Environment, Vol. 8 No. 4, pp. 270-291, doi: 10.1108/SASBE-09-2018-0045.
Lamb, K. (2018), “Grenfell tower public inquiry rule-witness statement”, available at: https://assets.grenfelltowerinquiry.org.uk/HAR00010419_Witness Statement of Kevin Lamb %28Harley Facades%29 %28Signed%29.pdf (accessed 28 November 2021).
Lane, B. (2018a), “Grenfell tower- fire safety investigation: phase 1 report - section 2 conclusions and next steps”, available at: https://assets.grenfelltowerinquiry.org.uk/documents/Dr%20Barbara%20Lane%20report%20-%20section%202%20%28Phase%201%20-%20supplemental%29.pdf (accessed 28 November 2021).
Lane, B. (2018b), “Grenfell Tower - fire safety investigation: phase 1 - Section 8 - the external wall- materials and construction”, available at: https://assets.grenfelltowerinquiry.org.uk/documents/Dr%20Barbara%20Lane%20report%20-%20section%208%20%28Phase%201%20-%20supplemental%29.pdf (accessed 28 November 2021).
Lane, B. (2018c), “Grenfell tower - fire safety investigation: phase 1 report - section 11: construction of the external walls”, available at: https://assets.grenfelltowerinquiry.org.uk/documents/Dr%20Barbara%20Lane%20report%20-%20section%2011%20%28Phase%201%20-%20supplemental%29.pdf (accessed 28 November 2021).
Lane, B. (2018d), “Grenfell tower - fire safety investigation, phase 1 report - section 4: overview of building works at Grenfell tower”, available at: https://assets.grenfelltowerinquiry.org.uk/documents/Dr%20Barbara%20Lane%20report%20-%20section%204%20%28Phase%201%20-%20supplemental%29.pdf (accessed 28 November 2021).
Lane, B. (2018e), “Grenfell tower - fire safety investigation: phase 1 report- section 10”, available at: https://assets.grenfelltowerinquiry.org.uk/BLAS0000010_Section%2010%20Barbara%20Lane%20Supplemental%20Phase%201%20Report_1.pdf (accessed 28 November 2021).
Liker, J.K. (2020), The Toyota Way: 14 Management Principles from the World's Greatest Manufacturer, 2nd ed., McGraw-Hill Education, New York, 978-1-260-46851-9.
Lu, W., Tan, T., Xu, J., Wang, J., Chen, K., Gao, S. and Xue, F. (2020), “Design for manufacture and assembly (DfMA) in construction: the old and the new”, Architectural Engineering and Design Management, Vol. 17 Nos 1-2, pp. 77-91, doi: 10.1080/17452007.2020.1768505.
Lydon, G.P., Caranovic, S., Hischier, I. and Schlueter, A. (2019), “Coupled simulation of thermally active building systems to support a digital twin”, Energy and Buildings, Vol. 202, pp. 1-19, 109298, doi: 10.1016/j.enbuild.2019.07.015.
McKenna, S.T., Jones, N., Peck, G., Dickens, K., Pawelec, W., Oradei, S., Harris, S., Stec, A.A. and Hull, T.R. (2019), “Fire behaviour of modern façade materials – understanding the Grenfell Tower fire”, Journal of Hazardous Materials, Vol. 368, pp. 115-123, doi: 10.1016/j.jhazmat.2018.12.077.
Meacham, B.J. and McNamee, M. (2020), Fire Safety Challenges of Green Buildings, Fire Protection Research Foundation, Quincy, MA, available at: https://www.nfpa.org/∼/media/Files/News%20and%20Research/Fire%20statistics%20and%20reports/Building%20and%20life%20safety/RFGreenBuildings2020.pdf (accessed 28 November 2021).
Meadows, D.H. (2008), Thinking in Systems: A Primer, Earthscan, London, 978-1-84407-726-7.
Montali, J. (2019), Digitized Engineering Knowledge for Prefabricated Façades, PhD thesis, University of Cambridge. doi: 10.17863/CAM.37905.
Montali, J., Overend, M., Pelken, P.M. and Sauchelli, M. (2018), “Knowledge-Based Engineering in the design for manufacture of prefabricated façades: current gaps and future trends”, Architectural Engineering and Design Management, Vol. 14 Nos 1-2, pp. 78-94, doi: 10.1080/17452007.2017.1364216.
NASA (2021), “MAPTIS: materials and processes technical information system”, available at: https://maptis.nasa.gov/Features#NASAmsd (accessed 28 November 2021).
New South Wales Department of Planning Industry and Environment (2019), “Guide for the assessment of buildings with combustible cladding”, available at: https://www.planning.nsw.gov.au/-/media/Files/DPE/Guidelines/Policy-and-legislation/guide-for-the-assessment-of-buildings-with-combustible-cladding-2019-09-05.pdf (accessed 28 November 2021).
Nguyen, K.T.Q., Navaratnam, S., Mendis, P., Zhang, K., Barnett, J. and Wang, H. (2020), “Fire safety of composites in prefabricated buildings: from fibre reinforced polymer to textile reinforced concrete”, Composites Part B: Engineering, Vol. 187, 107815, doi: 10.1016/j.compositesb.2020.107815.
O'Connor, J.T. and Koo, H.J. (2020), “Analyzing the quality problems and defects of design deliverables on building projects”, Journal of Architectural Engineering, Vol. 26 No. 4, 04020034, doi: 10.1061/(ASCE)AE.1943-5568.0000432.
O'Connor, J.T. and Koo, H.J. (2021), “Proactive design quality assessment tool for building projects”, Journal of Construction Engineering Management, Vol. 147 No. 2, pp. 1-11, 04020174, doi: 10.1061/(ASCE)CO.1943-7862.0001980.
Opus 2 International - Official Court Reporters (2020a), “Grenfell tower inquiry, day 85- kingspan evidence 9 december 2020”, available at: https://assets.grenfelltowerinquiry.org.uk/documents/transcript/Transcript%209%20December%202020.pdf (accessed 28 November 2021).
Opus 2 International - Official Court Reporters (2020b), “Grenfell tower inquiry-day 63”, available at: https://assets.grenfelltowerinquiry.org.uk/documents/transcript/GTI - Day 63.pdf (accessed 28 November 2021).
Pasini, D., Caffi, V., Daniotti, B., Lupica Spagnolo, S. and Pavan, A. (2017), “The INNOVance BIM library approach”, Innovative Infrastructure Solutions, Vol. 2 No. 15, pp. 1-9, doi: 10.1007/s41062-017-0062-y.
Peter, S., DeLone, W.H. and McLean, E.R. (2013), “Information systems success: the quest for the dependent variable”, Journal of Management Information Systems, Vol. 29 No. 4, pp. 7-61, doi: 10.2753/MIS0742-1222290401.
Potton, E. and Sutherland, N. (2020), “Grenfell tower fire: background”, available at: https://commonslibrary.parliament.uk/research-briefings/cbp-8305/ (accessed 28 November 2021).
Royal Institute of British Architects (2020), “The RIBA plan of work 2020”, doi: 10.4324/9780429347177-2 (accessed 28 November 2021).
Söderberg, R., Wärmefjord, K., Carlson, J.S. and Lindkvist, L. (2017), “Toward a Digital Twin for real-time geometry assurance in individualized production”, CIRP Annals - Manufacturing Technology, Vol. 66 No. 1, pp. 137-140, doi: 10.1016/j.cirp.2017.04.038.
Spearpoint, M., Fu, I. and Frank, K. (2019a), “Façade fire incidents in tall buildings”, CTBUH Journal No. 2, pp. 34-39, 2019, available at: https://www.ctbuh.org/resources/papers/4063-Journal2019_Issue%20II_FacadeFireIncidents.pdf (accessed 28 November 2021).
Spearpoint, M., Fu, I. and Frank, K. (2019b), CTBUH Facade Data set. Tall Building Facade Fire Incident Database, available at: https://ofrconsultants.com/tall-building-fire-incident-database (accessed 28 November 2021).
Stec, A.A., Dickens, K., Barnes, J.L.J. and Bedford, C. (2019), “Environmental contamination following the Grenfell Tower fire”, Chemosphere, Vol. 226, pp. 576-586, doi: 10.1016/j.chemosphere.2019.03.153.
Tassey, G. (2017), “The roles and impacts of technical standards on economic growth and implications for innovation policy”, Annals of Science and Technology Policy, Vol. 1 No. 3, pp. 215-316, doi: 10.1561/110.00000003.
Torero, J.L. (2018), “Grenfell tower: phase 1 report”, available at: https://assets.grenfelltowerinquiry.org.uk/documents/Professor%20Jose%CC%81%20L.%20Torero%20expert%20report.pdf (accessed 28 November 2021).
Vezzoli, C., Ceschin, F., Osanjo, L., M'Rithaa, M.K., Moalosi, R., Nakazibwe, V. and Diehl, J.C. (2018), Design for Sustainability: An Introduction, Designing Sustainable Energy for All. Green Energy and Technology, Springer, Cham, pp. 103-124, doi: 10.1007/978-3-319-70223-0_5.
White, N. and Delichatsios, M. (2015), “Fire statistics”, Fire Hazards of Exterior Wall Assemblies Containing Combustible Components, SpringerBriefs in Fire, Springer, New York, NY, pp. 17-24. doi: 10.1007/978-1-4939-2898-9_4.
Whyte, J. (2019), “How digital information transforms project delivery models”, Project Management Journal, Vol. 50 No. 2, pp. 177-194, doi: 10.1177/8756972818823304.
Wright, D. and Ceroni, M. (2017), “Systems thinking for design”, in Egenhoefer, R.B. (Ed.), Routledge Handbook of Sustainable Design, Routledge, London, pp. 13-26, ISBN 978-1-315-62550-8.
Yin, R.K. (2016), Qualitative Research from Start to Finish, 2nd ed., The Guilford Press, New York, NY, 9781462517978.
Yin, R.K. (2018), Case Study Research and Applications: Design and Methods, 6th ed., SAGE, Los Angeles, CA, 9781506336169.